Keywords
Abstract
The study of high-energy materials based on poly nitrogen- and nitrogen-oxygen-containing heterocycles is one of the most important and relevant modern interdisciplinary research areas at the intersection of organic and physical chemistry and materials science. Among such heterocycles, 1,2,4-oxadiazole ring is a rather interesting building block for the synthesis of new energetic compounds. Although the chemistry of 1,2,4-oxadiazoles has been developed for more than 100 years, high-energy materials based on these heterocycles have only recently become known and are currently one of the "hot spots" in this field of science. This review systematizes recently published methods for the synthesis and features of the reactivity of 1,2,4-oxadiazole-based energetic compounds. Mono- and bis(1,2,4-oxadiazoles) as well as structures containing other azoles such as pyrazole, 1,2,5-oxadiazole, 1,3,4-oxadiazole, 1,2,3-triazole, 1,2,4-triazole and tetrazole are considered. For the series of structurally similar compounds, their physicochemical properties are summarized and the factors affecting a particular parameter are discussed.
Bibliography — 123 references.
1. Introduction
The current state of scientific and technological development requires an ongoing search for materials with improved application properties. Recently, this problem has become particularly acute in the field of polynitrogen and nitrogen-oxygen energetic compounds, since traditional high-energy materials often prove unsuitable for solving new breakthrough scientific and technological challenges[1-8]. This is primarily due to the increased impact sensitivity of the most effective compounds and their non-compliance to modern requirements for energy efficiency and environmental safety. Moreover, the production of highly sensitive energetic substances inevitably entails an increase in anthropogenic risks, which is unacceptable for the development of advanced technologies in the XXI century[9-12]. Therefore, the search for an optimal balance between the physicochemical properties of energetic compounds and their impact sensitivity, taking into account the environmental safety of the synthesis methods, remains a pressing challenge.
The use of heterocyclic compounds as a synthetic platform for the construction of high-energy structures has significant advantages over the use of hydrocarbon frameworks. Such compounds have a high enthalpy of formation, higher density and high nitrogen content, which allows to reduce the amount of oxidant used in energetic compositions. At the beginning of the XXI century, a large number of high-energy materials based on various heterocycles were synthesized, new synthetic methods for their design have emerged, and nowadays, the trend in this field has completely shifted towards the application of heterocyclic derivatives[13-24]. In particular, 1,2,5-oxadiazole (furazan) and its N-oxide (furoxan) have the highest enthalpy of formation among isomeric oxadiazoles, which explains the extensive study of their derivatives as energetic materials of the new generation[25, 26]. It was shown that the combination of different azole cores in a molecule provides balanced properties of the compound[27-34]. It is important to note that the introduction of the 1,2,4-oxadiazole moiety into the molecule is expected to increase the thermal stability of the compound as well as to reduce the impact sensitivity, i.e. in this case, a balance between safety and efficiency of the material is achieved. For this reason, an optimal way to design energetic materials is to combine the 1,2,4-oxadiazole ring with other azoles and various explosophoric substituents (−NO2, −ONO2, −N−NO2 , −N=N−, −N=N(O), −N3 , −CN, −C(NO2)3).
The synthetic approaches to several energetic 1,2,4-oxadiazole derivatives are highlighted in a small review published in 2022[35]. However, since no comprehensive systematization of data on such derivatives has been carried out, the purpose of the present work was to summarize data on the synthesis and properties of high-energy compounds based on 1,2,4-oxadiazole. It considers both compounds based on mono- and bi(1,2,4-oxadiazoles) and structures containing other azoles, in particular pyrazole, 1,2,5-oxadiazole, 1,3,4-oxadiazole, 1,2,3-triazole, 1,2,4-triazole and tetrazole, and systematizes their physicochemical and energetic performance. It is important to emphasize that the 1,2,4-oxadiazole ring has only recently been considered as a valuable building block in the design of energetic materials. As a result, almost all of the information on this topic presented in this review has been published over the last 15 years, indicating the extreme relevance of this research area.
2. Energetic mono- and bi(1,2,4-oxadiazoles)
Energetic compounds based on 1,2,4-oxadiazole are generally under-represented in the literature compared to 1,2,5-oxadiazole-based structures, since the former compound has a relatively low enthalpy of formation (100 kJ mol–1) in the series of isomeric nitrogen-containing heterocycles, whereas the enthalpies of formation of furazan and furoxan are 216 and 226 kJ mol–1, respectively[36]. Nevertheless, examples of energetic compounds containing only 1,2,4-oxadiazole as the explosophoric group have been reported.
Thus, in 2012, diaminoglycoluril (1) was used as a starting material to produce 5-guanidino-3-nitro-1,2,4-oxadiazole (2), which then gave rise to a variety of energetic compounds (Scheme 1)[37]. Attempts to nitrate compound 2 with concentrated nitric acid in the absence of any additives led only to the isolation of the corresponding salt 3. At the same time, the use of a nitrating mixture consisting of sulfuric and nitric acids yielded 5-amino-3-nitro-1,2,4-oxadiazole (4), while the system HNO3 – P2O5 produced 5-nitramino derivative 5, isolated both in the free form and in the form of salt 5a. In addition, the Mannich reaction of 1,2,4-oxadiazole 2 with tert-butylamine gave compound 6, the substitutive nitration of which with 100% HNO3 and P2O5 produced nitramine 7, while the use of 100% HNO3 in the presence of NH4Cl led to the isolation of the product of substitutive nitrolysis 8.
The difference in the action of different nitration systems on compound 8 should also be noted. The treatment of this compound with a mixture of 100% HNO3 and P2O5 leads to oxidation to nitramine 7, and in the presence of the system 100% HNO3 – Ac2O, it undergoes cleavage to give oxadiazole 4 in low yield. Among the resulting compounds, 5-nitramino-3-nitro-1,2,4-oxadiazole (5) demonstrates detonation performance comparable to that of hexogen (cyclotrimethylene trinitramine, RDX) with comparatively lower impact sensitivity. Fu et al.[37] hypothesized that the nitro-1,2,4-oxadiazole core incorporated onto the molecule could improve the thermal stability of such a compound while reducing its impact sensitivity.
Based on 5-nitramino-3-nitro-1,2,4-oxadiazole (5) and guanidinium cations (Cat), a series of guanidinium energetic salts 5b – d was obtained (Scheme 2, Table 1)[38], among which salt 5b showed the best detonation performance (D = 9319 m s–1; P = 37.6 GPa), while maintaining high thermal stability (Tdес = 258 °C).
Acylation of 3,5-diamino-1,2,4-oxadiazole (9) with ethyl chloroformate yields the product 10, the subsequent nitration of which gives the compound 11 as the single tautomer, the mononitration product being formed exclusively[40]. Further reaction with hydrazine hydrate affords the salt 12a, which is converted to 3-amino-5-nitramino-1,2,4-oxadiazole (12) on acidification (Scheme 3). Ammonium and hydroxylammonium salts 12b,c were obtained by the reaction of compound 12 with an appropriate base in methanol. Hydrazinium salt 12a, with detonation performance comparable to that of hexogen (D = 8897 m s–1; P = 30.6 GPa) and lower sensitivity (IS = 20 J, FS (friction sensitivity) = 240 N), is the most promising among the resulting structures (Table 2)[40].
Chaves et al.[41] studied the reactivity of 3,5-diamino-1,2,4-oxadiazole (9) in the Mannich condensation with trinitroethanol, since the trinitroethyl moity can significantly increase the oxygen balance of the compound. It was found that only 3-amino group of the heterocycle undergoes this reaction to give structure 13. An attempt to use 2,2-dinitro-2-fluoroethanol in this process failed; the reaction product 14 was obtained only in trace amounts (Scheme 4). A complete characterization of the properties makes it possible to compare compounds 13 with hexogen, the former having a slightly better oxygen balance (–21.3% vs –21.6% for hexogen).
An accessible way of introducing a gem-dinitromethyl unit into an energetic compound is by oxidative nitration of the chloroxime group followed by the in situ reduction of the resulting chlorodinitromethyl intermediate.
When treated with an orthoester, diaminoglyoxime 15 is cyclized to (5-methyl-1,2,4-oxadiazolyl)amidoxime 16, and further diazotization in hydrochloric acid affords chloroxime 17, which is nitrated to give 5-methyl-3-(chlorodinitromethyl)-1,2,4-oxadiazole (18)[42]. The chlorodinitromethyl derivative 18 is then reduced with potassium iodide to produce the corresponding salt 19a. Further acidification of salt 19a generates compound 19, which is neutralized, without isolation, with nitrogenous bases to prepare a series of energetic salts 19b – h (Scheme 5). Such salts show good thermal stability, satisfactory impact and friction sensitivities (IS > 40 J, FS = 144 – 324 N) and superior detonation performance (D = 7845 – 8245 m s–1; P = 22.9 – 28.4 GPa) compared to similar properties of trotyl (D = 7303 m s–1; P = 21.3 GPa) (Table 3)[42].
A structurally similar chlorodinitromethyl derivative was obtained by nitration of compound 20 in a mixture of trifluoroacetic anhydride (TFAA) and CHCl3; further treatment of the intermediate 21 with potassium iodide and acidification gave 3-dinitromethyl-1,2,4-oxadiazole 22. Subsequent neutralization produced a series of salts 22b – d (see Scheme 5)[43].
A series of trinitromethyl-substituted 1,2,4-oxadiazoles were obtained from amidoxime 23 (Scheme 6). In the reaction of substrate 23 with diphosgene, an oxadiazolone moiety is formed and ethyl-2-(4,5-dihydro-1,2,4-oxadiazol-5-one-3-yl)acetate (24) is obtained, whereas the reaction with acid chlorides gives the open-chain intermediates 25 and 26, and subsequent heating of the reaction mixture yields 1,2,4-oxadiazole ring in the corresponding oxadiazole acetic acid derivatives 27 and 28. Acids 29 – 31, obtained via further saponification, were nitrated, and trinitromethyl products 32 and 33 were isolated from compounds 29 and 30 respectively, while attempts to nitrate the diacid 31 lead to the decomposition of the starting compound[44].
The oxadiazolone derivative 24 is further alkylated with dimethyl sulfate and ethyl bromoacetate to give the compounds 34 and 35, which were hydrolyzed to give the corresponding acids 36 and 37[44]. The nitration of the acid 37 delivers the trinitromethyl derivative 38, while the diacid 36 decomposes under these conditions. Nitration of the oxadiazolylacetic acid esters 24, 27 and 28 also yields the corresponding dinitro derivatives 39 – 41, which are converted into the salts 39a,b, 40a and 41a when reacted with potassium or barium hydroxide or when treated with an ammonia solution in methanol (Scheme 7). Trinitromethyl derivatives 32, 33 and 38 have high positive oxygen balance and detonation performance (D = 8779 – 8804 m s–1, P = 33.1 – 34.6 GPa), comparable with those of pentaerythritol tetranitrate (PETN): D = 8525 m s–1, P = 31.6 GPa (Table 4).
The reaction between ethyl 3-imino-3-ethoxypropanoate hydrochloride 42 and cyanamide in toluene produced N-cyanoimidate 43, which gave ethyl amino(1,2,4-oxadiazolyl) acetate in a similar manner. Depending on the order in which the reactants are mixed in this reaction, either the 5-amino isomer 44 or the 3-amino isomer 45 is formed[45]. Subsequent oxidation of amine 44 with potassium permanganate in acidic medium gives the corresponding azo derivative 46, further nitration of which leads to the cleavage of the C – N bond to furnish 3-(dinitromethyl)-1,2,4-oxadiazol-5-one in the form of the ammonium salt 47a (neutral compound 47 is obtained when treating salt 47a with hydrochloric acid in acetone). At the same time, oxidation of the regioisomeric 3-amino-1,2,4-oxadiazole 45 followed by nitration affords bis(dinitromethyl) derivative 48 retaining the structure of the oxadiazole ring. The treatment action of compound 48 with bases leads to salts 49a,b containing the dinitromethyl anion, and the neutral dinitromethyl derivative 49 is obtained upon acidification of salt 49a in acetone (Scheme 8).
The hydrazinium salt 49b has a rather high density of 1.78 g cm–3 and detonation performance comparable to that of hexogen (D = 8724 m s–1; P = 33.2 GPa) (see Table 4)[45]. Unfortunately, the original publication provided no data on the yields of compounds 44, 45, 48, 49 and 49b. Nitration of ammonium salt 49a gives compound 50, containing six nitro groups and thereby having positive oxygen balance (+6.9% to CO2) and detonation performance comparable to that of hexogen (D = 8722 m s–1, P = 33.2 GPa)[46].
Diaminoglyoxime 15, which can be cyclized to give both mono- and bi(oxadiazole) structures, is an important starting material for the synthesis of energetic 1,2,4-oxadiazole-based compounds. For example, condensation of diaminoglyoxime with diethyl malonate produces diethyl 2,2'-{[3,3'-bis(1,2,4-oxadiazole)]-5,5'-diyl}diacetate (51), which is further treated with a nitrating mixture to give diethyl 2,2'-{[3,3'-bis(1,2,4-oxadiazole)]-5,5'-diyl}bis(2,2-dinitroacetate) (52). The reaction of compound 52 with nitrogen-containing bases offers the potential for the synthesis of energetic dinitromethyl-1,2,4-oxadiazole salts 53a – j (Scheme 9)[47]. Hydroxylammonium salt 53b has the highest density (1.95 g cm–3) among the above compounds (Table 5).
Hydrolysis of diester 51 produces the corresponding diacid 54; attempts to crystallize it from DMF led to its decarboxylation to give dimethyl derivative 55. Substitutive nitration of diacid 54 proceeds as completely as possible to afford bi(trinitromethyl-1,2,4-oxadiazole) 56. It should be noted that nitration of the diamonium salt 53 with NO2BF4 also gives compound 56, but in lower yield[48]. The reaction of salt 53a with 1-fluoro-4-chloromethyl-1,4-diazoniabicyclo[2.2.2]octane bis(tetrafluoroborate) (trade name Selectfluor®) proceeds differently depending on the solvent: carrying out the process in water leads to the cleavage of the bond between the oxadiazole ring and the dinitromethyl anion to form bis(oxadiazolone) 57, whereas running the reaction in acetonitrile leads to the incorporation of the fluorine atom into the dinitromethyl anion to provide bis(fluorodinitromethyl) derivative 58[49]. It is also worth noting that the introduction of the fluorine atom instead of one of the nitro groups significantly increases the thermal stability of the compound and reduces its friction sensitivity (see Table 5).
The reaction of amidoxime 23 with oxalyl chloride gives diethyl 2,2'-{[5,5'-bis(1,2,4-oxadiazole)]-3,3'-diyl}diacetate 59, which is isomeric to compound 51. Its subsequent hydrolysis leads to a diacid 60, the nitration of which affords 3,3'-bis(trinitromethyl)-5,5'-bis(1,2,4-oxadiazole) 61, the regioisomer of structure 56[50]. When comparing the properties of the two regioisomers, compound 61 was found to have a slightly higher density, a higher decomposition temperature (142.8 °C) and friction insensitivity (Scheme 10).
Compound 57 was aminated to give structure 62, the nitration of which gives dinitramide 63, which has high density (1.932 g cm–3), moderate thermal stability and detonation characteristics comparable to those of octogen (D = 9106 m s–1, P = 36.6 GPa)[51]. When treated with bases, nitramide 63 delivers a number of energetic salts 63a – e (Scheme 11). Hydroxylammonium salt 63e shows detonation velocity and pressure (D = 9507 m s–1, P = 40.1 GPa) comparable to those of isowurtzitane CL-20, and also high density (1.929 g cm–3) (Table 6).
Cyclization of diaminoglyoxime 15 with chloroxoethyl acetate gave bis(oxadiazole) derivative 64, which was further hydrolyzed to give alcohol 65. Subsequent nitration of compound 65 led to bis(1,2,4-oxadiazole)bis(methylene)dinitrate 66 (Scheme 12). By optimizing the synthesis conditions, the yield of the target compound was improved more than five-fold[52]. The resulting material is proposed to be used as a potential melt-castable explosive as well as an energetic plasticizer.
The reaction of diaminoglyoxime 15 with chloroacetyl chloride followed by boiling in dimethylformamide gave bis(chloromethyl-1,2,4-oxadiazole) 67, after which the chlorine atoms in the product were successfully replaced by azido groups to furnish bis(azidomethyl-1,2,4-oxadiazole) 68 (Scheme 13)[53]. Similarly, bis(amidoxime)furoxan 69 was converted to the dichloromethyl derivative 70, which was further reduced with tin(II) chloride in hydrochloric acid to give furazan 71. Treatment of compounds 70 and 71 with sodium azide in acetonitrile provided the corresponding azidomethyl derivatives 72 and 73. These compounds are liquid at room temperature, have high enthalpies of formation (1072.9 – 1079.9 kJ mol–1), and also show lower impact sensitivity compared to trinitrotoluene, while having similar detonation properties (D = 7672 – 8071 m s–1; P = 22.7 – 26.1 GPa) (Table 7).
Johnson et al.[54] managed to combine the 1,2,4-oxadiazole ring and the nitratomethyl-substituted isoxazole core in a molecule. In an attempt to obtain the starting compound 74 by cyclization of diaminoglyoxime with triethyl orthoformate, a mixture of mono- (74) and bicyclization products (75) was isolated in the form of a co-crystallisate. However, treatment of such a co-crystallisate with sodium nitrite in concentrated HCl leads to the precipitation of pure hydroxymoyl chloride 20. The formation of the isoxazole ring in structure 76 is achieved by [3 + 2]-cycloaddition of propargyl alcohol to nitrile oxide generated from hydroxymoyl chloride 75 (Scheme 14). Further nitration of the hydroxymethyl group in compound 76 gives the product 77, which has detonation properties comparable to those of trinitrotoluene (D = 7087 m s–1, P = 21.0 GPa) and also melts without decomposition at 76.6 °C (Tdec = 184.5 °C) (Table 8), allowing its use as a melt-castable explosive.
Cao et al.[55] proposed a convenient synthetic approach to 5,5'-diamino-3,3'-bi(1,2,4-oxadiazole) based on the cyclization of diaminoglioxime 15 and trichloroacetic anhydride (TCAA) to form the compound 78 and the substitution of the amino functionality by the trichloromethyl group using ammonia solution in methanol. Subsequent nitration of the diaminooxadiazole 79 gives dinitramine 80, which can be neutralized with various nitrogen-rich bases to deliver a series of energetic salts 80a – g (Scheme 15). Among these compounds, the hydroxylammonium salt 80b has the best detonation performance (D = 8916 m s–1, P = 36.2 GPa), exceeding that of hexogen (see Table 8).
A similar synthetic scheme was used to obtain energetic structures of a new type, consisting of two 1,2,4-oxadiazole rings bridged with a methylene unit so as to desensitize the resulting structure (Scheme 16)[56]. Replacing the trichloromethyl group in the derivative 81 with an amino group affords methylenebis(amino-1,2,4-oxadiazole) 82. When compound 82 was nitrated with nitric acid, the authors failed to extract dinitramine 83 from the aqueous fraction. However, the use of N2O5 as a nitrating agent greatly simplifies isolation and purification of product 83, the neutralization of which produced a series of energetic salts[56]. Such compounds have good thermal stabilities and moderate impact sensitivities. Among salts 83a – f, the hydrazinium salt 83b has the highest detonation performance (D = 7962 m s–1, P = 27.1 GPa) (Table 9).
An important class of high-energy materials are compounds containing an azo functionality (–N=N–) which acts as a linker to connect azole rings in the molecule. It was shown that the presence of the azo moiety significantly increases the enthalpy of formation of the molecule and reduces its impact sensitivity. For example, Thottempudi et al.[57] report the synthesis of azo-coupled 5-amino-1,2,4-oxadiazole 84 using sodium dicyanoamide (Scheme 17). However, an attempt to nitrate the amino groups of compound 84 with nitric acid in acetic anhydride only led to azobis(1,2,4-oxadiazolone) 85 isolated in 54% yield. It was suggested that the intermediate dinitramide decomposes during the aqueous work-up of the reaction mixture, with the nitro group being replaced by the hydroxyl group, thereby affording compound 85. Due to the presence of the N – H bond in the azobis(oxadiazolone) structure, 85 reacts with basic amines to give a series of energetic salts 85a – c in high yields. These compounds show lower impact sensitivities than trinitrotoluene (Table 10).
Triazene (−NH – N=N) and amino (–NH–) functionalities can also be used as bridging groups for azoles, as the presence of the N – H linkage makes them excellent precursors for energetic salts. Oxidation of 5-amino-3-nitro-1,2,4-oxadiazole (4) with sodium dichloroisocyanurate (SDIC) gave a mixture of amino- and triazene-bridged derivatives 86a and 87a (Scheme 18). After separation of the mixture, individual products were subjected to the two-step cation metathesis, which provided a series of the corresponding energetic salts 86b – d and 87b – d[58]. Detonation performance parameters of these compounds (D = 7701 – 8542 m s–1; P = 24.2 – 29.4 GPa) are comparable to those of trinitrotoluene (see Table 10) and these molecules show much lower impact sensitivities. Nitration of both amino groups in the azo compound 84 furnished the dinitramide azobis(oxadiazole) 88, and subsequent metathesis with nitrogen-containing bases gave rise to a series of energetic salts 88a – h (Scheme 19). Among these derivatives, the hydroxylammonium salt 88a is the most promising compound, combining high detonation properties (D = 9243 m s–1; P = 39.2 GPa) with acceptable thermal stability (Tdec = 169 °C) and sensitivity (Table 11)[59].
In addition to azo functionalities and methylene bridges, it is possible to use an ethene unit as a linker between nitrogen-containing heterocycles[60]. In this case, the overall planar structure of the target compound makes it possible to reduce its impact and friction sensitivities compared to similar structures containing an azo group, as well as to increase its density. The addition of hydroxylamine to fumaronitrile 89 afforded the starting compound 90, which was cyclized with trifluoro- and trichloroacetic anhydrides to give products 91 and 92 respectively. Replacement of the CCl3 moiety with an amino group by reaction with gaseous NH3 yields compound 93, and further nitration and metathesis with bases provided dinitramine 94 and its energetic salts 94a – d (Scheme 20). Among the structures obtained, the salts 94c,d have acceptable sensitivities (IS = 15 – 20 J, FS = 360 N) and good detonation performance (D = 8778 – 9305 m s–1, P = 32.2 – 33.4 GPa) (Table 12), which allow their use as energetic materials. It is also worth noting that an attempt to oxidize the amino groups in compound 93 with hydrogen peroxide resulted in the decomposition of the starting compound[61].
3. 1,2,4-Oxadiazole/pyrazole-based energetic compounds
The pyrazole ring has long attracted the attention of researchers due to its high thermal stability, as well as the possibility of chemical modification and introduction of various functional groups into the molecule, including amino, nitramino and nitro groups[62, 63]. In particular, a number of energetic compounds containing pyrazole and 1,2,4-oxadiazole heterocycles have been synthesized from commercially available 1H-pyrazole-3-carboxylic acid (95). The polycyclic precursors 96 and 97 were obtained by cyclization of the acid chloride 98 with pyrazolyl amidoxime and diaminoglyoxime respectively. Further nitration of the pyrazole ring at the C(3) and C(4) positions was carried out under different conditions. For example, when treated with excess nitric acid and acetic anhydride, N-nitro derivatives are formed which, when heated to 140 °C in benzonitrile, are rearranged to the corresponding 3-nitro derivatives 99 and 100 (Scheme 21)[64]. Further nitration of compounds 99 and 100 gives dinitropyrazoles 101 and 102. On the other hand, the use of a nitrating mixture consisting of sulfuric and nitric acids leads to 4-nitro derivatives 103 and 104, which were further nitrated with a mixture of nitric acid and acetic anhydride to yield compounds 105 and 106 containing a nitro group at the heterocyclic nitrogen atom. Among the compounds obtained, products 101 and 102 have comparable detonation velocities and pressures (D = 8685 – 8741 m s–1, P = 33.4 – 34.0 GPa), while being less sensitive to impact and friction (Table 13), and structures 105 and 106 have the best detonation performances (D = 8867 – 8904 m s–1, P = 34.5 – 35.1 GPa).
In 2022, high-energy molecules were synthesized containing three different azoles: pyrazole, furazan and 1,2,4-oxadiazole[65]. The first cyclization of amidoxime 107 with 1H-pyrazole-3-carbonyl chloride affords the tricyclic derivative 108, which is oxidized with a mixture of 98% sulfuric acid and 30% hydrogen peroxide to give the nitro compound 109 in 68% yield. A sequential introduction of nitro groups into the pyrazole ring is then carried out using different synthetic strategies. First, the dinitro derivatives 110 and 111 (the latter was not isolated in the individual state) were prepared and subjected to additional nitration to isomers 112 and 113 (Scheme 22)[65]. Compound 113 has a high density (1.876 g cm–3), good detonation velocities and pressures (D = 9094 m s–1, P = 36.7 GPa) exceeding those of hexogen, as well as acceptable sensitivity values (IS = 24 J, FS = 300 N) and satisfactory thermal stability (Tdec = 265 °C) (Table 14).
Since the NH functionality in compound 113 is rather acidic due to the presence of two electron-withdrawing nitro groups in the pyrazole ring, an attempt was made to convert this compound to the salt form by the action of a weak base (aqueous ammonia solution). Instead, a chemoselective substitution of the nitro group in the furazan ring by an amino group was observed, and energetic salts were obtained by nitration of product 114 with fuming nitric acid followed by the treatment with an aqueous ammonia solution, hydrazine hydrate or hydroxylamine (Scheme 23). The salts 115a – c have detonation properties corresponding to the neutral compound (D = 8618 – 8996 m s–1, P = 32.0 – 36.3 GPa).
A series of isomeric 3,5-bis(nitropyrazolyl)-1,2,4-oxadiazoles were prepared using a strategy of acylation of the corresponding amidoximes 116 with pyrazole carboxylic acid chlorides 117 in acetonitrile in the presence of triethylamine to give derivatives 118 and subsequent closure of the 1,2,4-oxadiazole ring in boiling acetic acid. This furnished the target products 119a–i. The combination of regioisomeric nitropyrazoles as starting compounds allows a wide range of isomeric structures to be obtained in sufficiently high yields (Scheme 24)[66]. Such compounds have high decomposition temperatures and moderate to high densities. In the range of isomeric structures, heterocycle 119b has the highest density (1.804 g cm–3) and excellent thermal stability (Tdec = 343 °C), as well as detonation properties comparable to those of trotyl (D = 7670 m s–1, P = 27.5 GPa) (Table 15).
A similar synthetic methodology has been used for the oxadiazole ring closure in compounds 120a,b[67]. Further oxidation of the amino group in the 1,2,5-oxadiazole moiety of tricyclic energetic structures 121a,b yields nitro derivatives 122a,b or azo compounds 123a,b (Scheme 25)[67]. These heterocycles have detonation properties (D = 7800 – 8100 m s–1, P = 30 – 32 GPa) exceeding those of TNT, while exhibiting comparable impact sensitivities (Table 16).
Energetic structures containing methylene-bridged 5-nitramino-3,4-dinitropyrazole and 5-nitrosamino-1,2,4-oxadiazole moieties were constructed using a similar synthetic approach (Scheme 26)[68]. The nitro group at the pyrazole ring in heterocycle 124 is replaced by an amino group when treated with ammonia to give compound 125, and further nitration affords dinitramine 126. The action of alkali on compounds 125 and 126 affords the corresponding potassium salts 127 and 128. A study of the physicochemical properties shows that compound 128 is extremely impact sensitive and has good detonation performance (D = 8059 m s–1, P = 30.9 GPa) (Table 17), which allows it to be considered as a primary explosive.
Previously, the same research group[69] obtained similar energetic compounds differing in the set of substituents in polynitropyrazole (Scheme 27). The addition of hydroxylamine to nitrile 129 leads to the corresponding amidoxime 130, and the 5-amino-1,2,4-oxadiazole ring in compound 131 is constructed via cyclization of substrate 130 with cyanogen bromide in the presence of sodium bicarbonate. Attempted nitration of the amino group at the pyrazole ring in compound 131 gave unexpectedly the internal diazonium salt 132 instead of nitramine, and the action of 30% hydrogen peroxide in sulfuric acid allowed the chemoselective oxidation of the amino group at the pyrazole ring to afford three nitropyrazoles 133. Subsequent oxidative coupling afforded a tetracyclic azo derivative 134 and nitration with nitric acid furnished nitramine 135. It is worth noting that when neutralizing compound 135 with excess ammonia, a nucleophilic substitution of one of the nitro groups in the pyrazole rings occurs, leading to the salt 136. Among the structures obtained, the azo compound 134 has the best thermal stability (Tdec = 250 °C), good detonation performance (D = 8897 m s–1, P = 35.2 GPa) and moderate sensitivity to mechanical stimuli (IS = 10 J, FS = 120 N) (Table 18). (Heterocycle 135 has detonation characteristics comparable to those of hexogen (D = 9014 m s–1, P = 36.7 GPa), but is extremely impact sensitive (IS = 1.5 J).
4. 1,2,4-Oxadiazole/1,2,5-oxadiazole-based energetic compounds
Energetic compounds based on a combination of 1,2,4- and 1,2,5-oxadiazole rings have been thoroughly studied. The introduction of the 1,2,5-oxadiazole core into the molecule makes it possible to significantly increase the enthalpy of formation, detonation rate and density[70], so its combination with the 1,2,4-oxadiazole ring, which reduces impact sensitivity, is a promising approach to the design of new high-energy materials.
An extremely valuable and commonly used starting compound for the preparation of energetic materials is 4-amino-3-furazancarboxamidoxime (107), which is readily synthesized from commercially available malononitrile[71]. Condensation with diethyl oxalate gives 3,3'-bis(4-aminofurazan-3-yl)-5,5'-bis(1,2,4-oxadiazole) 137, oxidation of the amino groups provides dinitro derivatives 138 (Scheme 28)[71]. Similar cyclization with cyanogen bromide leads to 3-(4-aminofurazan-3-yl)-5-amino-1,2,4-oxadiazole 139, which further undergoes chemoselective oxidation to form the nitrofurazan moiety in compound 140. Furthermore, the reaction between amidoxime 107 and 3-amino-4-cyanofurazan 141 in the presence of zinc chloride as a Lewis acid opens the way to the synthesis of 3,5-bis(4-amino-1,2,5-oxadiazol-3-yl)-1,2,4-oxadiazole 142[72, 73], which is further oxidized with 70% hydrogen peroxide to the dinitro derivative 143 or nitrated. In the latter case, the dinitramino derivative 144 is formed, which is isolated as ammonium and hydrazinium salts 144a,b.
Nitration of diamine 139 with nitric acid and subsequent treatment of the dinitramine intermediate with gaseous ammonia gives ammonium salt 145a, which is further subjected to metathesis with silver nitrate and nitrogen-containing bases to afford the energetic salts 145b – g (Scheme 29)[73]. Compounds 144a,b, 145a – g show good thermostabilities, have a high enthalpies of formation (Table 19) and acceptable densities. These salts have lower impact and friction sensitivities compared to hexogen, and salt 145c has the best detonation performance in this series of compounds (D = 9046 m s–1, P = 37.4 GPa).
Aminofurazanylamidoxime was also used as a starting material for the synthesis of a number of tetracyclic azo derivatives combining 1,2,4-oxadiazole and furazan cores. Oxidation of oxadiazolylaminofurazans 139 and 146 with potassium permanganate in hydrochloric acid gave the azo compounds 147 and 148 (Scheme 30)[74]. Subsequent nitration of the heterocycle 148 in acetic acid furnishes the dinitramine derivative 149. Oxidation of compound 150 leads to the azo compound 151, and oxadiazolylaminofurazan 152 gives the product 153. These compounds decompose at temperatures above 240 °C (Table 20). It is worth noting that heterocycle 149 has a high density (2.12 g cm–3) and enthalpy of formation (1038 kJ mol–1), as well as excellent detonation properties (D = 10114 m s–1, P = 46.1 GPa), exceeding those of CL-20 and octogen, but the sensitivity of this compound is slightly lower than that of CL-20.
An azo group can be further reduced or oxidized to extend a pool of energetic structures. In order to study the influence of the azo group on the performance of energetic materials, a series of molecules based on 1,2,4-oxadiazole and furazan, bearing different linker groups, have been synthesized (Scheme 31)[75]. The oxidative coupling of diamine 139 gives the azo structure 148 comprising two azo-bridged furazan rings. The subsequent nitration of compound 148 with nitric acid in acetic anhydride leads to the sequential formation of the first one and then another oxadiazolone moiety to give structures 154 and 155 respectively. It should be noted that in an attempt to obtain the hydrazinium salt 155, the reduction of the N=N double bond to a single bond is observed to produce the hydrazo derivative 156a, which on acidification gives a neutral compound 156. The oxidation of diamine 139 leads to heterocycle doubling to form the azo structure 157, which, under similar conditions, is converted to the dioxadiazolone derivative 158. Energetic salts 155a – d, 158a–d were derived from the above compounds and their energetic performance was studied in detail. The library of energetic salts was further extended in a patent[76], but the authors provide no information on the yields of compounds 155e – k. These compounds have high densities and enthalpies of formation, good detonation performance; they are also thermally stable (Tdec = 204 – 322 °C) and insensitive to friction and impact (Table 21). The neutral azo derivative 155 decomposes at 322 °C, this temperature being significantly higher than that of the hydrazine derivative 156 (271 °C) and the azo derivative 158 (307 °C). The same regularity is followed in the series of the corresponding salts, showing the advantage of the azo group present in the structure of the energetic compound in terms of thermal stability. Compound 155 also gave rise to a number of energetic salts insensitive to impact and friction. The azoxy derivative 158 shows superior detonation characteristics (D = 8844 m s–1, P = 34.4 GPa) than azo or hydrazo compounds; a similar pattern is observed when comparing the salts with corresponding cations.
Various N-alkyl moieties can be used as linkers for the azole rings. For example, the amino derivative 146 reacts with formaldehyde to give the product 159. Oxidation of substrate 146 to nitro derivative 160 and subsequent nucleophilic substitution of the nitro group by ethylenediamine affords the diamine-bridged heterocycle 161 (Scheme 32). These compounds were nitrated with a mixture of acetic anhydride and 100% nitric acid to give the dinitramine derivatives 162 and 163, which were further reacted with hydrazine hydrate to undergo the opening of the oxadiazole ring and to afford the corresponding products 164 and 165[77].
These compounds are characterized by moderate thermal stability, acceptable density, high values of enthalpy of formation and detonation velocity, and low impact sensitivity. It should be noted that for compounds 164 and 165, which are free from the 1,2,4-oxadiazole ring, the values of density and thermostability are significantly reduced (Table 22).
Amidoxime 107 reacts with acetamide at high temperature to give 3-amino-4-(5-methyl-1,2,4-oxadiazol-3-yl)furazan 150[78]. Subsequent reaction of the latter with trinitroethanol gives the product 166, which is further nitrated with a mixture of 100% HNO3 and Ac2O to produce the polynitro derivative 167. Compound 150 was also nitrated with fuming nitric acid and its further reaction with a number of bases gave salts 168a – i (Scheme 33). It should be noted that when the ether solution of compound 168 is washed with brine during the purification process, the sodium salt 168d is formed, the properties of which have been studied together with the corresponding organic salts. The resulting compounds are insensitive to impact and friction (except for nitramine 167), and the hydroxylammonium salt 168a has the best detonation performance in this series D = 8545 m s–1, P = 30.2 GPa), close to that of hexogen (Table 23)[73, 78].
Compound 137 undergoes the Mannich reaction with trinitroethanol and then the NH groups of the intermediate 169 can be nitrated to form compound 170 (Scheme 34)[79]. In addition, nitration of the amino groups in compound 137 gives dinitramine 171. Treatment of the latter with bases, both directly and after the intermediate formation of the silver salt 172a, produces energetic salts 171a – q[79, 80]. These salts are thermally stable (Table 24), in contrast to their precursor 171, which has a decomposition temperature of as low as 84 °C.
He et al.[81] explored optimum conditions for cyclocondensation as an approach to compound 173, which is nitrated to afford the nitramine derivative 174 and reacts with bases to give the energetic salts 174a – c (Scheme 35). The densities of the products exceed those of hexogen and the detonation performance (D = 8652 – 9064 m s–1, P = 32.8 – 38.0 GPa) are close to that of octogen (see Table 24). It should be noted that these salts are relatively insensitive to mechanical stimuli, making them potential secondary explosives.
Oxadiazolone 175 was obtained via oxidative cleavage of the 5-methyl-1,2,4-oxadiazole moiety in compound 150[82]. Oxadiazolone 175 was further subjected to azo coupling, nitration and oxidation under various conditions to give the corresponding products 176, 177 and 178. The Mannich reaction of compound 175 with trinitroethanol produces the derivative 179, and neutralization of the heterocycles 177 and 178 with nitrogen-containing bases furnishes the energetic salts 177a – c and 178a – c (Scheme 36)[82]. Among these compounds, the neutral compounds have densities higher than those of the corresponding salts (Table 25), and compound 179, which has an acceptable sensitivity (IS = 16 J, FS = 240 N) with good detonation performance (D = 8637 m s–1; P = 32.2 GPa), proved to be the closest to practical use as an alternative to hexogen.
According to literature data[83-85], energetic salts of nitrogen-containing heterocycles usually have higher thermal stability compared to neutral precursors. Based on these considerations, we studied the properties of a series of nitrogen-rich energetic salts 180a – f, obtained by chemoselective nitration of compound 139 with subsequent neutralization of the reaction product 180 (Scheme 37)[86]. Only one amino group in the furazan heterocycle is nitrated, since the strong inductive effect of the oxygen atom of the 1,2,4-oxadiazole ring significantly reduces the reactivity of the second amino group in the molecule. Salts 180a – f have considerably higher decomposition temperatures (175−235 °C), than the neutral compound 180 (111 °C), and also excellent detonation performance (D = 8191 – 8838 m s–1, P = 26.0 – 33.3 GPa) (Table 26).
One way to improve the oxygen balance in the target energetic molecules is the introduction of a nitramine or nitroether moiety into nitrogen-rich heterocyclic frameworks. This approach was tested on the example of compound 181 obtained by the reaction of 2-oxoethyl-2-chloroacetate with amidoxime 107[87]. Direct nitration of the heterocycle 181 produces nitramine 182, which is converted to the ammonium salt 182a (Scheme 38). At the same time, the preliminary hydrolysis of the substrate 181 to the compound 183, followed by nitration, gives the nitroether 184, which is further converted into the salt forms 184a – c by two-step metathesis. Compound 181 is also subjected to the oxidative coupling with simultaneous hydrolysis of the acetyl groups to afford the azo structure 185, which is converted into nitroether 186 when treated with nitric acid. The resulting compounds show good thermal stabilities (except for the neutral compound 184) and good detonation performance (D = 7809 – 8822 m s–1, P = 27.2 – 35.2 GPa) (Table 27).
Similarly, the azido derivative 187 was derived from aminofurazanylamidoxime 107, which was further subjected to nitration with the reaction product being isolated in the form of silver salt 187a, followed by metathesis to afford salts 187b–e (Scheme 39)[88]. The authors also carried out the oxidation of the aminofurazan functionality in compound 187 to the nitrofurazan moiety. Oxidation product 188 is a liquid at room temperature and has acceptable detonation properties (D = 8290 m s–1; P = 28.6 GPa) (see Table 27).
1,2,5-Oxadiazole N-oxide (furoxan) containing a ‘hidden nitro group’ due to the presence of two active oxygen atoms, which makes it possible to improve the oxygen balance of the compound and increase the density and detonation efficiency of the material, is a valuable structural building block for the design of energetic materials[25, 89].
In 2015, the conditions for the synthesis of the 1,2,4-oxadiazole ring attached to furoxan were optimized. 3-Methyl-4-cyanofuroxane 189 was converted to amidoxime 190, which was further condensed with an excess of trimethyl orthoformate in the presence of scandium trifluoromethanesulfonate (triflate, OTf) (10 mol.%) as a Lewis acid (Scheme 40)[90]. The reaction proceeds in 1 min to give rather high yield of the product 191 (86%). Using the same procedure, dicyanofuroxane 192 gave bis(amidoxime) 193 and 3,4-bis(1,2,4-oxadiazol-3-yl)furoxane 194 in 82% yield. This method was scaled up in 2019 and optimized by replacing the Lewis acid with BF3 · Et2O, resulting in an increase in the overall product yield[91]. Compound 194 has a high density (1.79 g cm–3) and enthalpy of formation (357 kJ mol–1), as well as good detonation performance (P = 24.1 GPa, D = 7577 m s–1) and low sensitivity to mechanical (IS > 34.7 J, FS > 360 N) and electrostatic (ESD > 0.25 J) stimuli.
A similar method was used to prepare the tricyclic furoxan derivative 195 containing directly linked 5-amino-1,2,2-oxadiazole rings[92]. The synthesis of bis(1,2,4-oxadiazol-3-yl) furoxan 195 was carried out via cyclization of bis(amidoxime) 193 with cyanogen bromide (Scheme 41). This procedure was modified in work[32], where the authors managed to increase the yield of the target product and studied its physicochemical and energetic properties. Compound 195 is characterized by high thermal stability, decomposing at 269 °C, and also has a detonation velocity and pressure (D = 7280 m s–1, P = 23.7 GPa) comparable to those of trotyl.
Chemoselective addition of hydroxylamine to dicyanofuroxan 192 gives amidoxime 196, and subsequent cyclization with cyanogen bromide delivers 4-(5-amino-1,2,4-oxadiazol-3-yl)-3-cyanofuroxane (197)[93]. Upon addition of sodium azide to cyanofuroxan in the presence of zinc bromide, tetrazole ring is formed in the structure 198, further nitration of which gives the nitramino derivative 199. The two-step synthesis of chloroxime 200 followed by nitration with nitric acid in trifluoroacetic anhydride affords a dinitromethyl derivative isolated as the potassium salt 201a. The corresponding energetic salts 199a – e and 201b,c were also obtained by the metathesis reaction (Scheme 42). (It should be noted that the metathesis of potassium salt 201a leads to a double salt with only 1 equiv. of potassium being substituted).
The salts 199a – c,e are characterized by a relatively higher thermal stability (179 – 234 °C) compared to the neutral compound 199, which decomposes at 121.2 °C (Table 28). In this series, the highest densities are observed for compounds 199 and 199c (1.86 and 1.84 g cm–3), while the hydrazinium salt 199b, despite its comparatively low density, has an extremely high enthalpy of formation (702.9 kJ mol–1) and consequently a high detonation velocity (D = 8713 m s–1, P = 29.7 GPa), comparable to that of hexogen. Salt 201a has the following detonation properties: D = 8076 m s–1, P = 29.8 GPa, exceeding similar parameters of lead azide (Pb(N3)2), and it is extremely sensitive to impact (IS = 3 J) but not to friction (FS = 60 H). This allows us to consider structure 201a as a potent ‘green’ alternative to toxic primary lead azide-based explosive compositions.
As mentioned above, the reactivity of the amino group in the 1,2,4-oxadiazole ring is often greatly reduced because of the lack of electron density due to the strong electron-withdrawing effect of the intracyclic oxygen atom[92]. Based on these data, we developed a method for the synthesis of tetracyclic energetic molecules containing 1,2,5- and 1,2,4-oxadiazole rings. First, cyclization of aminofuroxanylamidoxime 202 with cyanogen bromide gave 5-amino-3-(4-aminofuroxanil)-1,2,4-oxadiazole 203, in which the amino groups at the furoxan ring were selectively oxidized to give the product 204. Further oxidative coupling under the action of the KMnO4 – HCl system produced the tetracyclic azo derivative 205 (Scheme 43). Compounds 140 and 206 containing the furazan ring were obtained in a similar way[94, 95]. When comparing the energetic properties of the obtained products, it was found that the densities, enthalpyiesof formation and detonation performance of the azo derivatives were significantly superior to those of the precursors. When comparing furazan and furoxan derivatives, their N-oxides were found to have higher densities, enthalpies of formation and detonation properties than furazan derivatives bearing no N-oxide functionality. All the heterocycles obtained show acceptable impact and friction sensitivities. Compound 205 is less impact sensitive than hexogen, and its detonation performance parameters (D = 9666 m s–1, P = 42.8 GPa) are comparable to those of CL-20, which allows us to recommend this heterocycle as a promising explosive (Table 29).
The amino group at the furoxan ring of compound 203 can be also involved in the azo coupling to give the azo derivative 207, which was further nitrated with different reagents to give the oxadiazolone or nitraminooxadiazole derivatives 208 and 209 (Scheme 44)[96]. Two series of energetic salts (208a – c and 209a – c) were obtained by metathesis with different nitrogen-containing amines. Among these compounds, heterocycle 209 has the highest density (1.92 g cm–3) and high detonation performance (D = 9505 m s–1, P = 41.3 GPa), while having a sensitivity (IS = 4 J, FS = 80 N) comparable to that of CL-20 (see Table 29).
A similar strategy was used to synthesize tetracyclic azo derivatives bearing explosophoric substituents. The oxidative coupling of aminofurazan 210 followed by nitration of the azo structure 211 leads to a dinitromethyl derivative 212 which is further converted to the bis(dinitromethyl) ammonium salt 213a by the reaction with an aqueous ammonia solution[97]. An attempt to obtain the hydrazinium salt in a similar way resulted in a mixture of salts of azo (213b) and hydrazino derivatives (214a) and, using acetonitrile as a solvent, the azo group is reduced to the hydrazinium moiety and salt 214a is isolated in a good yield (Scheme 45). The pathway of the reaction of compound 213 with an aqueous solution of hydroxylamine depends on the amount of the reagent: with an equimolar amount of hydroxylamine, the hydroxylammonium salt 213c is formed, while using an excess leads to the complete destruction of the azo-bridge to afford the amino derivative 215a in the form of an ammonium salt in a mixture with the product 213c. Nitration of the ammonium salt 213a also gives the bis(trinitromethyl)azo compound 216 (Scheme 46)[98].
Using aminofuroxan 217 as a starting compound, an azo derivative 218 was obtained from which tetracyclic azo compounds 219 and 220 containing di- and trinitromethyl groups were synthesized similar to compounds 213 and 216[99]. It should be noted that, unlike the furazan-containing analogues, the synthesis of the energetic salts 219a – c produces no products of the azo group reduction (Scheme 47). The detonation performance parameters of all the above compounds are comparable to those of hexogen, and the bis(trinitromethyl) derivatives 216 (D = 9351 m s–1; P = 37.46 GPa) and 220 (D = 9411 m s–1; P = 39.0 GPa) show detonation velocities and pressures comparable to those of octogen (Table 30). The hydrazinium salt 214a is impact insensitive.
The library of energetic tetracyclic oxadiazoles has been extended by combining various explosophoric substituents with azo-bridged oxadiazole rings[100]. Addition of hydroxylamine to the cyano group of compounds 221 and 192 followed by condensation of the resulting amidoximes 222 and 196 with BrCN gave 4-azido-3-(5-amino-1,2,4-oxadiazolyl)furoxan 223 (see [101]) and 4-(5-amino-1,2,2-oxadiazolyl)-3-cyanofuroxan 197 respectively[93]. The target energetic azo-bridged tetracycles 224 and 225 were synthesized by oxidative coupling of compounds 223 and 197 using KMnO4 in hydrochloric acid (Scheme 48).
Azo structures 224 and 225 have high nitrogen and oxygen contents (>68%) and, due to the presence of different azole rings in the molecule, high enthalpies of formation (1720.0 and 1221.9 kJ mol–1), significantly exceeding those of hexogen and octogen (Table 31). In addition, the detonation parameters of these compounds exceed those of trinitrotoluene, while the first compound (D = 9090 m s–1, P = 37.4 GPa) is also superior to hexogen.
Using the precursors 226 – 228, Tang et al.[102] synthesized bicyclic 3-amino-1,2,4-oxadiazoles 229 – 231. The key step of the process is the oxidative cyclization of N-carbamoyl-substituted 1,2,5-oxadiazoles 232 – 234, initiated by PhI(OAc)2 (Scheme 49). The compounds 229 – 231 react differently with hydrazine monohydrate depending on their structure: furoxan 229 does not undergo this reaction, whereas furazan 230 gives the aminotriazole 235, and in the case of the tetracyclic azo compound 231, both the reduction of the azo-bridge and the opening of the aminooxadiazole ring to afford the heterocycle 236 are observed. The detonation performance of these compounds exceed that of trinitrotoluene (Table 32)[102].
An effective method for constructing an energetic molecule is the use of fused polyazole frameworks. Dou et al.[103] have succeeded in synthesizing energetic compounds based on imidazo[1,2-d][1,2,4]oxadiazole, which is additionally fused to the nitraminofurazan ring via the C – C bond. The key step in the synthesis is the annulation of 4-nitro-2-chlorimidazole to amidoxime 237 in the presence of triethylamine as a base (Scheme 50). Next, the amino group of intermediate 238 is nitrated. The product 239 and its energetic salts 239a – e have detonation properties comparable to those of hexogen (D = 8051 – 8682 m s–1, P = 25.5 – 32.8 GPa) (Table 33), being less impact sensitive.
5. 1,2,4-Oxadiazole/1,3,4-oxadiazole-based energetic compounds
Among isomeric azoles containing nitrogen and oxygen atoms, 1,3,4-oxadiazole has the lowest enthalpy of formation, and chemical modification of the substituents at the carbon atoms in the ring is rather difficult and requires strict conditions. Nevertheless, a number of energetic 1,3,4-oxadiazole-based compounds are known that combine low impact sensitivity with increased thermal stability, such as 5,5'-bis(2,4,6-trinitrophenyl)-2,2'-bi(1,3,4-oxadiazole) (TKX-55) with a decomposition temperature >335 °C[104]. The use of quantum chemical calculations using the Density Functional Theory (DFT) method[105] allowed us to determine the potential properties of compounds containing 1,2,4- and 1,3,4-oxadiazole rings. The calculated detonation parameters exceed those of hexogen, so that the incorporation of the 1,3,4-oxadiazole fragment into the energetic structure may help to achieve a balance between the stability of the compound and its detonation performance.
To date, a small number of energetic compounds containing 1,2,4- and 1,3,4-oxadiazoles with good detonation properties have been synthesized. For example, cyclization of diaminoglyoxime 15 with ethyl chlorooxoacetate gives bi(1,2,4-oxadiazole) 240, which is then sequentially reacted with hydrazine to give hydrazide 241 and with cyanogen bromide to give the tetracyclic structure 242. Further nitration and reaction with bases produces compound 243 and its salts 243a – c (Scheme 51). In this series, compound 243 has the highest density (1.90 g cm–3), comparable to that of hexogen. Among the salts, the hydrazinium salt 243c has the highest detonation velocity (D = 8648 m s–1) and the hydroxylammonium salt 243b has the highest detonation pressure (P = 31.7 GPa) (Table 34). The resulting structures show acceptable impact sensitivities (IS = 10 – 20 J, FS = 110 – 240 N), and together with high thermal stability and high detonation performance, they can be considered as a new generation of energetic materials[106].
Similarly, it is possible to carry out the sequential construction of isomeric oxadiazole rings in ethyl cyano formate 244[107]. Addition of hydroxylamine gives amidoxime 245, further cyclization with cyanogen bromide gives aminooxadiazole 246, which is further converted to hydrazide 247. Closure of the 1,3,4-oxadiazole ring affords compound 248, subsequent nitration gives dinitramine 249, and metathesis involving its produces a series of energetic salts 249a – d (Scheme 52). The study of the energetic properties of the above compounds shows that product 249 and its non-metallic salts 249b–d are significantly less sensitive to mechanical stimuli than hexogen, with comparable detonation parameters (D = 8080 – 8888 m s–1, P = 23.6 – 34.1 GPa) (Table 35)[107].
6. 1,2,4-Oxadiazole/1,2,3- (or 1,2,4)-triazole-based energetic compounds
The combination of 1,2,4-oxadiazole and triazole heterocycles is quite rare in energetic materials. In 2020, a number of structures based on the 1,2,3-triazole/1,2,4-oxadiazole combination were synthesized[108]. Using 5-amino-1H-1,2,3-triazole (250) as a starting compound, amidoxime 251 and bicyclic precursor 252 were obtained by a tandem addition– cyclization, followed by ammonolysis to provide 5-amino-3-(5-amino-1H-1,2,3-triazol-4-yl)-1,2,4-oxadiazole (253) (Scheme 53). Subsequent nitration with various reagents gives the mononitration product 254 and (mediated by carbamate 255) dinitration product 256, isolated in the form of the corresponding energetic salts 254a – c и 256a – d. The preliminary reaction of the heterocycle 253 with ethyl chlorophormate to form the structure 255 enables the amino group in the 1,2,4-oxadiazole ring, which reactivity is significantly reduced, to be nitrated. The resulting salts have high enthalpies of formation and acceptable impact sensitivities (Table 36). It was shown that the salts 254a – c bearing one nitramine group are more thermally stable but have lower detonation performance compared to the characteristics of the corresponding analogues 256a – d with two nitramine groups.
A similar strategy was used to obtain the 1,2,4-triazole isomer[109]. 5-Amino-3-cyano-1,2,4-triazole (257) was treated with hydroxylamine, the intermediate amidoxime 258 was then reacted with trichloroacetic anhydride to give the product 259. Further treatment with methanolic ammonia leads to the replacement of the trichloromethyl group with the amino group to give 5-amino-3-(5-amino-1H-1,2,4-triazol-3-yl)-1,2,4-oxadiazole (260). Nitration under different conditions gives mono- (261) and dinitration products (262), which are used to prepare a wide range of energetic nitrogen-containing salts 261a – n and 262a – n (the original publication provided no yields of these salts) (Scheme 54)[109]. In this series, products 262 and 262c have comparable detonation performance (D = 9087 – 9135 m s–1, P = 35.3 – 35.9 GPa) (see Table 36) and lower sensitivities (IS = 16 – 20 J, FS = 252 – 288 N), making them safer substitutes for hexogen and octogen.
Detailed studies of energetic compounds based on the combination of triazole and 1,2,4-oxadiazole heterocycles have not been carried out. Nevertheless, a number of compounds combining triazole and furazane rings, as well as explosophoric groups, in particular nitro and nitramino functionalities[110], have been described[111-114]. This approach to the design of nitrogen-containing high-energy structures is the most common and effective.
7. 1,2,4-Oxadiazole/tetrazole-based energetic compounds
Tetrazole stands out among energetic compounds due to its high nitrogen content and high enthalpy of formation (237 kJ mol–1)[115]. The combination of tetrazole and 1,2,4-oxadiazole in a molecule significantly improves the enthalpy of formation of the energetic material, and the additional introduction of a hydroxyl group into the tetrazole ring increases the density of the compound and improves its detonation performance[116]. In addition, deprotonation of hydroxytetrazole or tetrazole opens the way to the preparation of energetic salts. At present, only a few energetic compounds combining 1,2,4-oxadiazole and tetrazole rings are known. The first examples of such structures were described in 1989[117]. The authors found that heating azidoximes 263, derived from halooximes 264, in ether, leads to the closure of the tetrazole ring in compound 265 instead of the expected product of the azole-azole rearrangement 266 (Scheme 55).
Zhang et al.[118] reported examples of melt-castable energetic materials containing methylene-bridged 5-nitrotetrazole and 1,2,4-oxadiazole rings for the first time. The starting 2-(5-nitro-2H-tetrazol-2-yl)acetonitrile (267) reacts with hydroxylamine hydrochloride in the presence of sodium bicarbonate to give the compound 268 and then, depending on the electrophilic agent in choice, the closure of the 1,2,4-oxadiazole ring provides the products 269 – 271 (Scheme 56). These compounds have good thermal stabilities (Tdec > 240 °C) and detonation properties (D = 7271 – 7909 m s–1, P = 20.9 – 24.8 GPa), which exceed those of trinitrotoluene (Table 37).
In 2017, a method was developed to prepare energetic derivatives of 1,2,4-oxadiazole containing a directly linked hydroxytetrazole ring[71]. 3,3'-Bis(5-cyano-1,2,4-oxadiazole) (272) was obtained from bis(1,2,4-oxadiazole) 240 in two steps. Further addition of hydroxylamine and diazotization of the product in hydrochloric acid gives bis(chloroxime) 273. The final step of the process involves the replacement of the chlorine atom by an azide group followed by cyclization with hydrochloric acid in dioxane to give 3,3'-bis[5-(1-hydroxy-1H-tetrazol-5-yl)-1,2,4-oxadiazole] (274), which is converted to the salts 274а – е (Scheme 57). Similarly, di(cyano)oxadiazole 276 is obtained from ethyl cyanoformate via the intermediate diethyl 1,2,4-oxadiazole-3,5-dicarboxylate 275. The product (277) of the addition of hydroxylamine to the compound 276 in alcohol is further subjected to diazotization with subsequent replacement of the chlorine atom by an azide group and formation of diazidoxime 278. Cyclization of the latter using hydrochloric acid in dioxane gives 3,5-bis(1-hydroxy-1H-tetrazol-5-yl)-1,2,4-oxadiazole (279), which is converted to the ammonium salt 279a. Heterocycles 274, 274a, 279a are insensitive to impact, friction and electrostatic discharge, and the decomposition temperature of all these compounds, according to differential scanning calorimetry data, exceeds the similar parameter of hexogen[71].
Nitrogen-rich salts derived from compound 274 have been used to study the effect of the counter-ion on the density of the structure and crystal packing[119]. The density of such salts varies from 1.54 to 1.87 g cm–3, but their detonation properties have not been investigated (yields are also not given).
This synthetic methodology was developed in a study[43], where a series of high-energy compounds based on 1,2,4-oxadiazole containing various energetic substituents, including the 1-hydroxytetrazole moiety, were obtained. The interaction of chloroxime 20 with sodium azide gives azidoxime 280 and its subsequent cyclization in acidic medium leads to the target 5-(1,2,4-oxadiazol-3-yl)-1-hydroxytetrazole 281 (Scheme 58). Reaction with bases in alcohol gives a series of energetic salts 281a – c.
Compound 281 melts at 133 °C and decomposes at 143 °C, while its salts decompose without melting between 141 and 174 °C. The salts also show a detonation performance (D = 8576 – 8929 m s–1, P = 28.0 – 32.7 GPa) higher than that of hexogen, which allows to propose them as potential explosives. It should be noted that the neutral compound 281 is the most impact sensitive in this series (IS = 6 J, FS = 72 N) (see Table 38).
Dong et al.[120] explored two different strategies for the synthesis of bis[5-(tetrazol-5-yl)-1,2,4-oxadiazole] 282 from available reactants and obtained its energetic nitrogen-containing salts 282a – e. The method, based on the condensation of diaminoglyoxime with tetrazolylacetic acid 283 mediated by the intermediate acid chloride 284, gave the target product in lower yields and has a longer reaction time compared to the [3 + 2] cycloaddition to di(cyano)oxadiazole 272 (Scheme 59). The resulting compounds are sufficiently thermostable (Tdec > 216 °C) and their detonation properties (D = 7984 – 8112 m s–1, P = 22.8 – 25.6 GPa) are superior to those of trinitrotoluene (Table 39).
8. Compounds based on 1,2,4-oxadiazole and six-membered rings
The library of heterocycles used in the design of new-generation energetic materials is not limited to azoles. For example, studies[121, 122] showed the possibility of obtaining hybrid structures containing tetrazine, a nitrogen-rich six-membered heterocycle, together with 1,2,4-oxadiazoles (Scheme 60). Starting from tetrazine 285 and azidooxadiazole 286, a diazidooxadiazole derivative 287 was obtained and reaction with compound 2 gave the product 288.
Product 288 has an outstanding thermal stability (Tdec = 328 °C) and low impact sensitivity (IS = 70 J), and its detonation velocity and pressure (D = 8002 m s–1, P = 28.5 GPa) exceed similar parameters of trinitrotoluene and are only slightly lower than those of hexogen (Table 40).
Examples of the preparation of a variety of polynitro-3,5-diaryl-1,2,4-oxadiazoles are described and the influence of nitro groups in the aromatic ring on the energy parameters of a number of such compounds is estimated (Scheme 61)[123]. The reaction of mono- or dinitro-substituted aryl cyanides 289 with hydroxylamine hydrochloride in aqueous ethanol gives the corresponding amidoximes 290, then the mixture of the corresponding amidoxime and N-acylbenzotriazole in boiling dimethyl formamide or ethanol in the presence of triethylamine affords polynitro-3,5-diaryl-1,2,4-oxadiazoles 291. It was found that the density of the resulting structures correlated directly with the number of nitro groups and it was suggested that 3,5-bis(2,4,6-trinitrophenyl)-1,2,4-oxadiazole will have density and detonation characteristics similar to those of hexogen. However, the properties of the above structures have not been investigated.
9. Conclusion
To summarize, the analysis of literature data reveals a diversity of types of 1,2,4-oxadiazole-based energetic structures. In recent years, a large number of high-energy compounds have been obtained containing one or two 1,2,4-oxadiazole rings in combination with conventional explosophoric groups (nitro, azido, azo groups, etc.). It should be noted, however, that the variety of such structural combinations is very limited, leading researchers to look for other ways to create an ‘ideal’ energetic material. In this context, it is obvious that a promising trend is the combination of the 1,2,4-oxadiazole core with other known explosophoric heterocycles (pyrazole, triazole, tetrazole as well as isomeric oxadiazoles) in a molecule. Implementing such a synthetic strategy is largely combinatorial and usable for the preparation of energetic materials, not only based on 1,2,4-oxadiazole, leading to an avalanche-like increase in the number of publications on this subject and a simultaneous growth of competition in the scientific community for the priority obtaining of the most promising materials. A certain diversification of the 1,2,4-oxadiazole-based energetic bi- and polyheterocyclic structures synthesized so far should be noted: for example, combinations of the 1,2,4-oxadiazole ring with pyrazole or 1,2,5-oxadiazole cores are widely represented in the literature, whereas similar structures based on the combination of the 1,2,4-oxadiazole ring with triazole or 1,3,4-oxadiazole cores are very few in number. Obviously, this can be due to the inaccessibility of functional derivatives of 1,2,4-(or 1,3,4)-oxadiazole and 1,2,3-(or 1,2,4)-triazole, which will require the development of new synthetic methods to carry out such studies in the coming years.
One of the strategies of obtaining heterocyclic combinations based on 1,2,4-oxadiazole can also be considered as the synthesis of energetic salts derived therefrom, which are usually characterized by higher stabilities in comparison with neutral precursors. Despite the fact that the 1,2,4-oxadiazole ring is not capable of salt formation, the introduction of NH-azoles or functional groups with high acidity (e.g. dinitromethyl group) as substituents into such a molecule helps to obtain a wide range of energetic salts. Varying the cationic part in ionic structures allows to control the physicochemical properties of target high-energy materials, although it should be noted that they may have increased hygroscopicity.
In conclusion, it is important to point out that the literature sources systematized in this review have been published over the last 15 years, since earlier publications on the above subject are practically non-existent. This is due, first of all, to the fact that the 1,2,4-oxadiazole heterocycle has not been considered as an energetic group throughout the XX century, being surpassed by more potent derivatives based on tetrazole, 1,2,5-oxadiazole and tetrazine. However, modern trends in the development of energetic materials science dictate requirements for the balance of physicochemical properties of high-energy materials, i.e. the search for a less potent but more thermally stable and less sensitive compound. These criteria are largely met by the 1,2,4-oxadiazole-based systems, which already represent quite a promising class of energetic components. We have no doubt that the study of synthetic approaches for the design of energy-rich 1,2,4-oxadiazoles will be intensively developed in the coming years and will lead to the creation of new promising materials based thereon.
10. List of abbreviations and designations
Bt — benzotriazol-1-yl,
Cat — cation,
CL-20 — 2,4,6,8,10,12-hexanitro-2,4,6,8,10,12-hexaazaisowurtzitane,
D — detonation velocity,
DBU — 1,8-diazabicyclo[5.4.0]undec-7-ene,
DFT — density functional theory,
ESD — electrostatic discharge sensitivity,
FS — friction sensitivity,
\( \Delta H_{o}^f \) — standard enthalpy of formation,
HMX — octogen (cyclotetramethylenetrinitramine),
IS — impact sensitivity,
Oxone® — potassium peroxymonosulfate,
P — detonation pressure,
PETN — pentaerythritol tetranitrate,
PIDA — di(acetoxy)iodobenzene,
py — pyridine,
r — density,
RDX — hexogen (cyclotrimethylenetrinitramine),
SDIC — sodium dichloroisocyanurate,
Selectfluor® — 1-chloromethyl-4-fluoro-1,4-diazoniabi-cyclo[2.2.2]octane bis(tetrafluoroborate),
Tdec — decomposition temperature,
TCA — trichloroacetic acid,
TCAA — trichloroacetic anhydride,
TEA — triethylamine,
TFA — trifluoroacetic acid,
TFAA — trifluoroacetic anhydride,
TfO — trifluoromethanesulfonate (triflate),
TNT — trinitrotoluene,
Ts — p-toluenesulfonyl (tosyl).
References
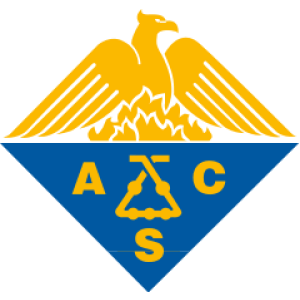
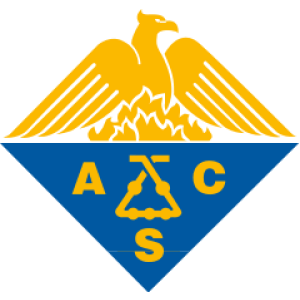
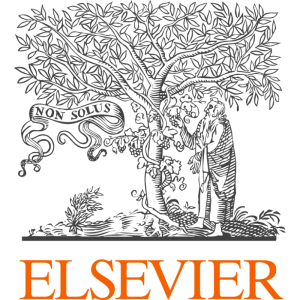
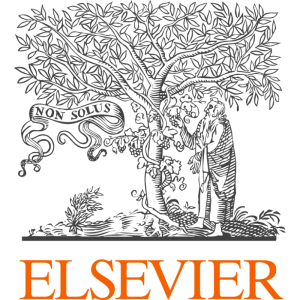
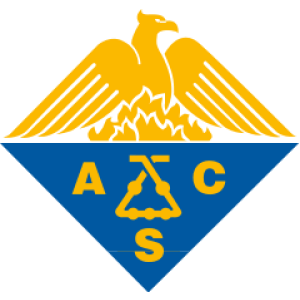
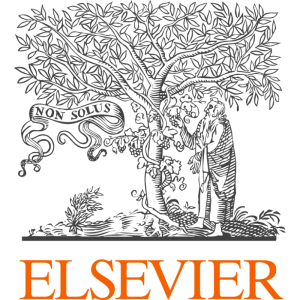
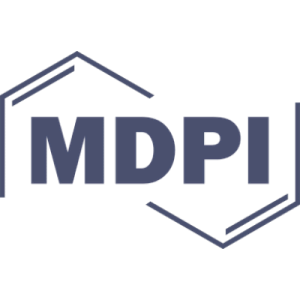
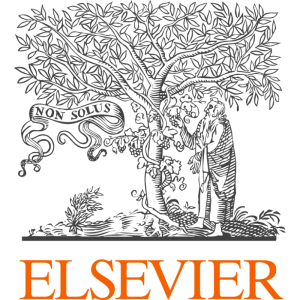
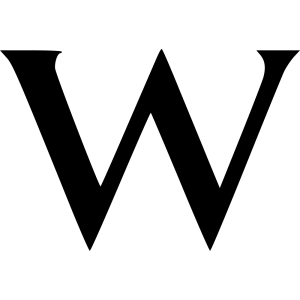
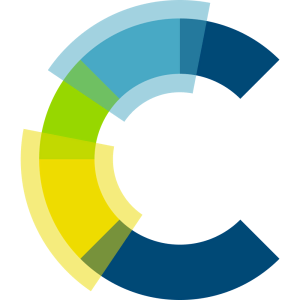
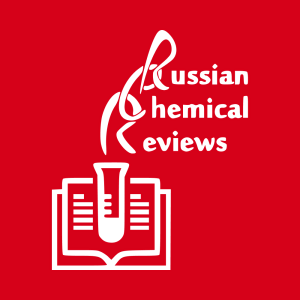
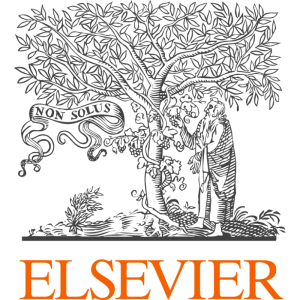
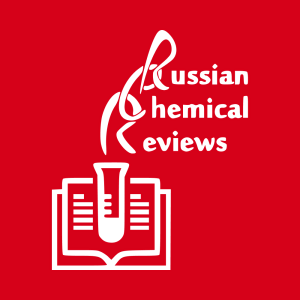
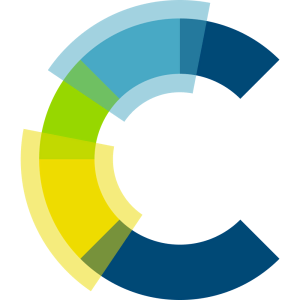
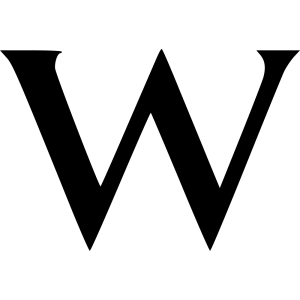
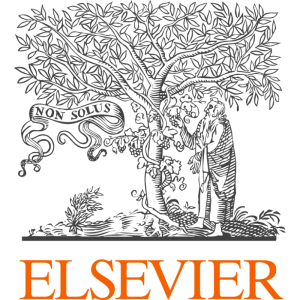
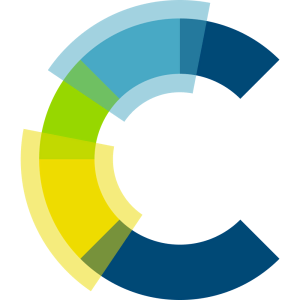
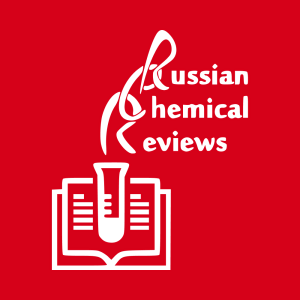
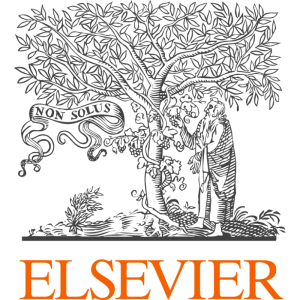
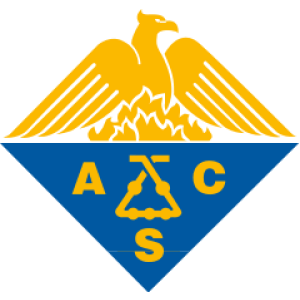
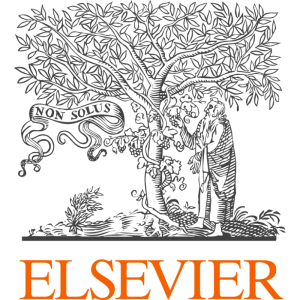
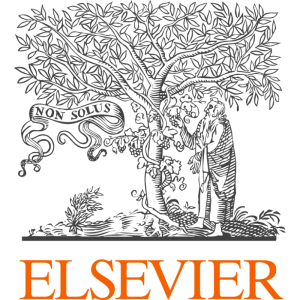
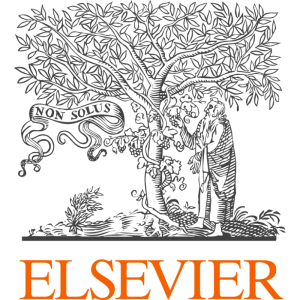
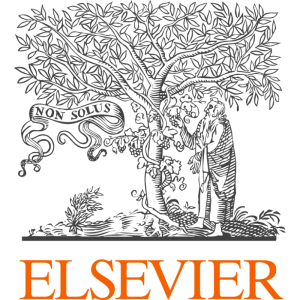
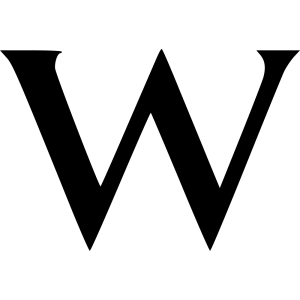
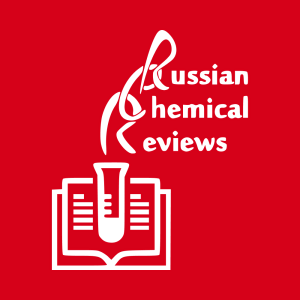
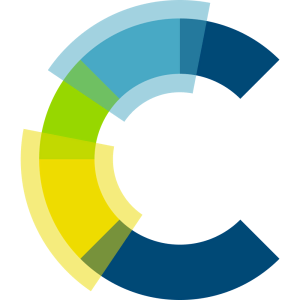
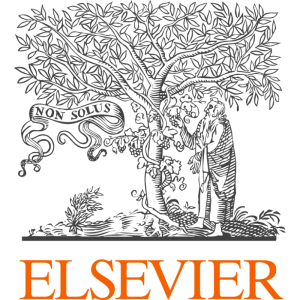
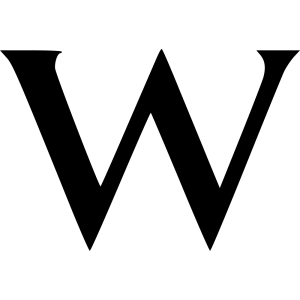
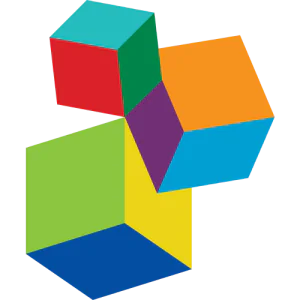
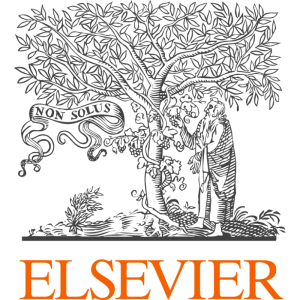
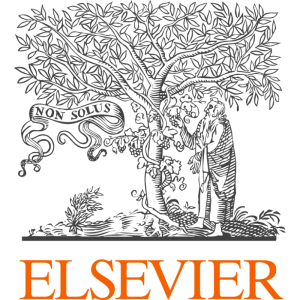
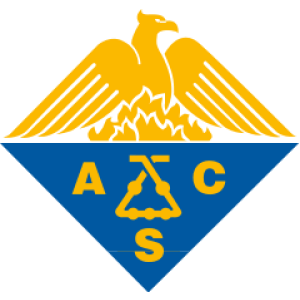
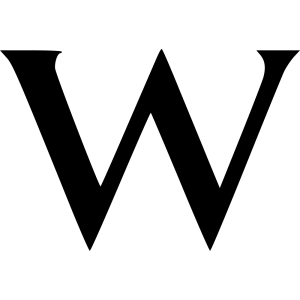
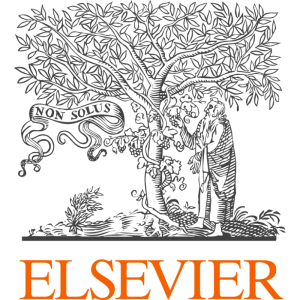
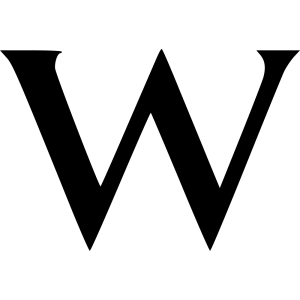
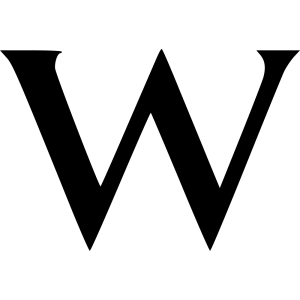
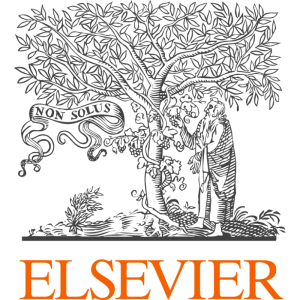
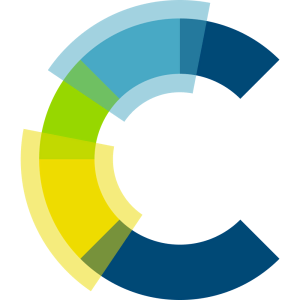
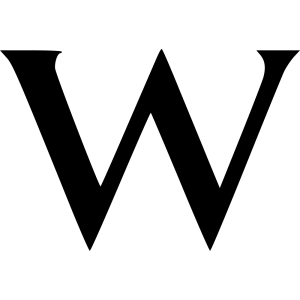
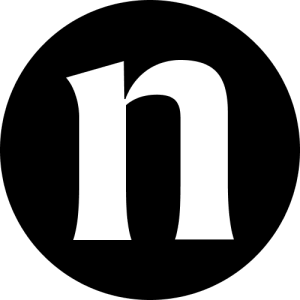
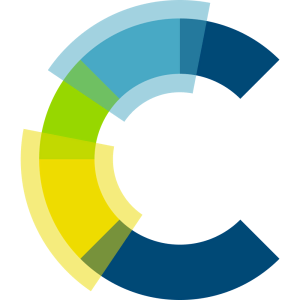
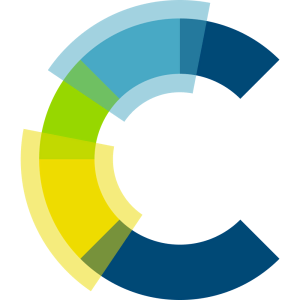
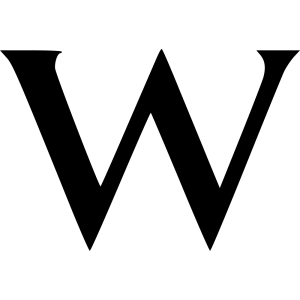
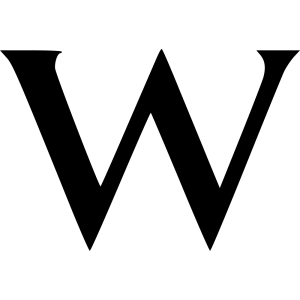
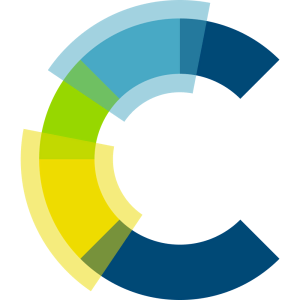
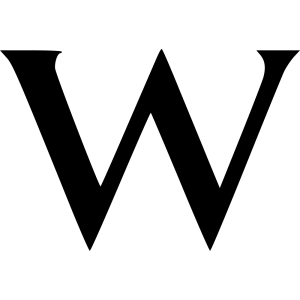
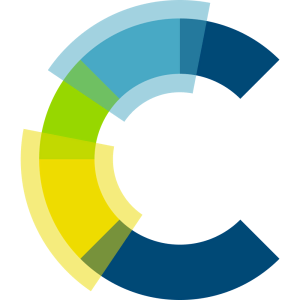
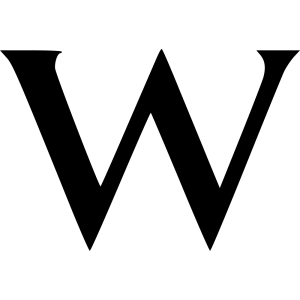
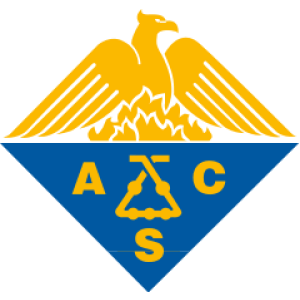
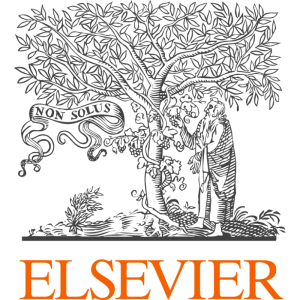
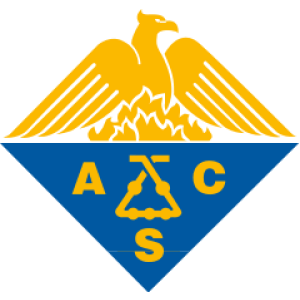
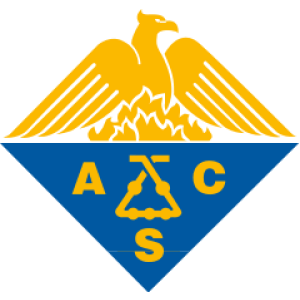
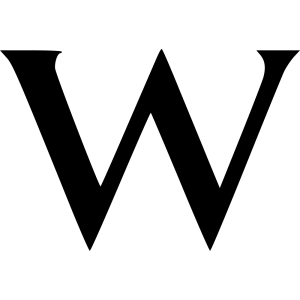
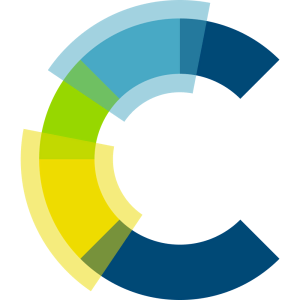
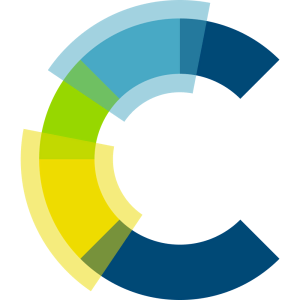
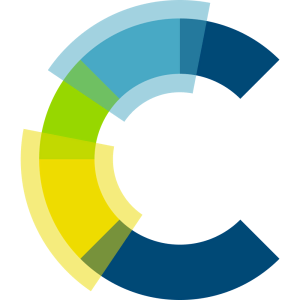
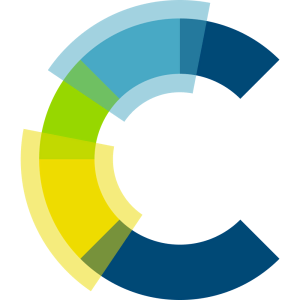
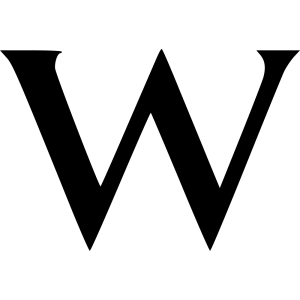
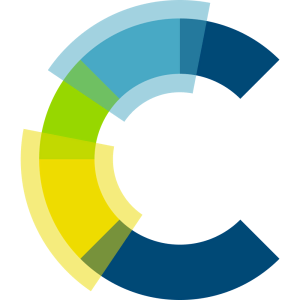
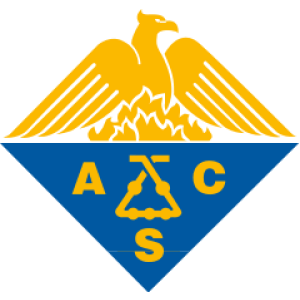
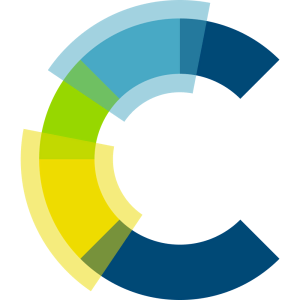
![3,6-Dinitropyrazolo[4,3-c ]pyrazole-Based Multipurpose Energetic Materials through Versatile N-Functionalization Strategies](/storage/images/resized/bRyGpdm98BkAUYiK1YFNpl5Z7hPu6Gd87gbIeuG3_small_thumb.webp)
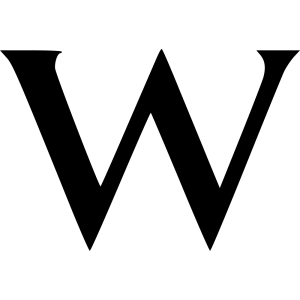
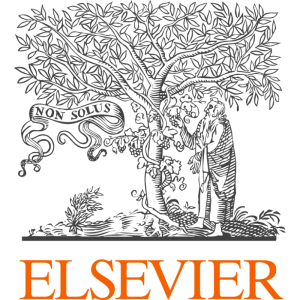
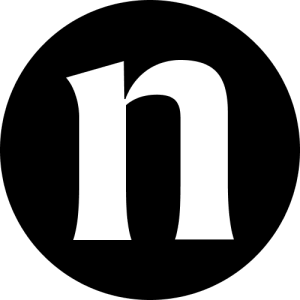
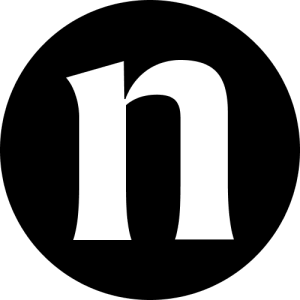
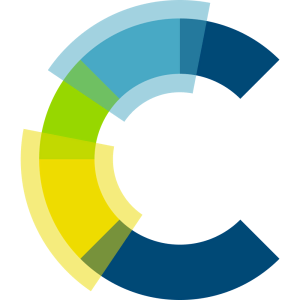
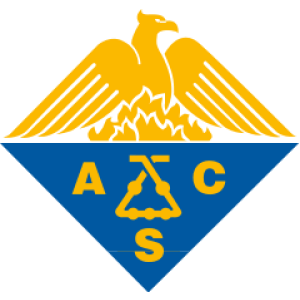
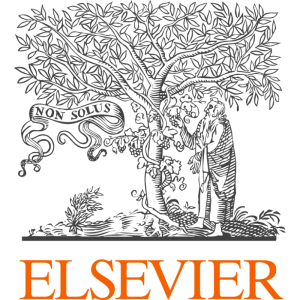
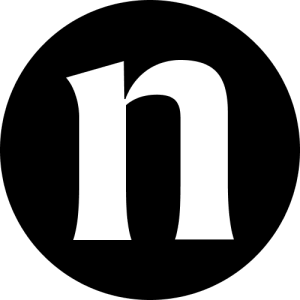
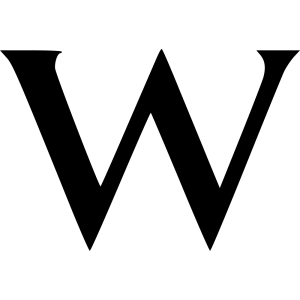
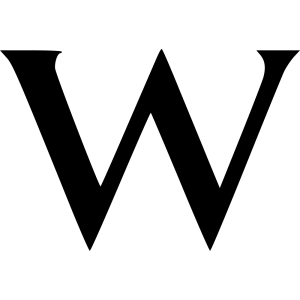
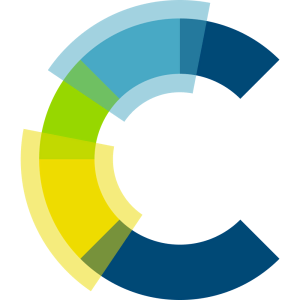
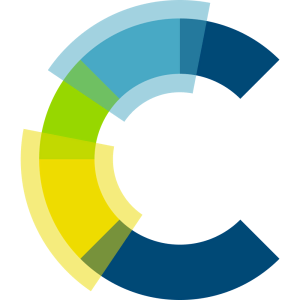
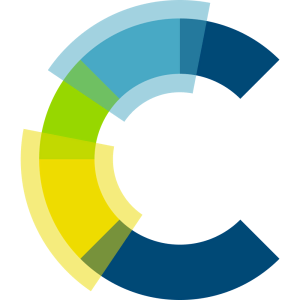
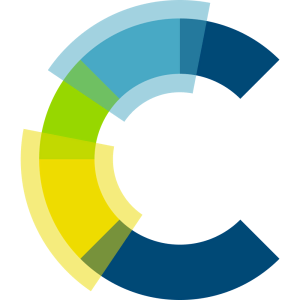
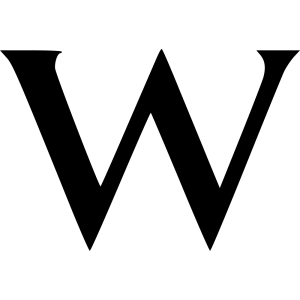
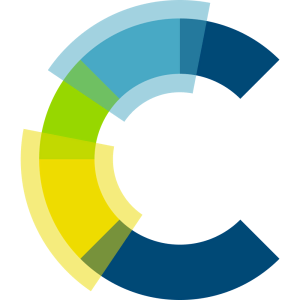
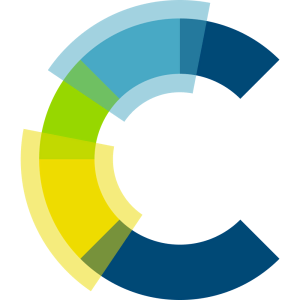
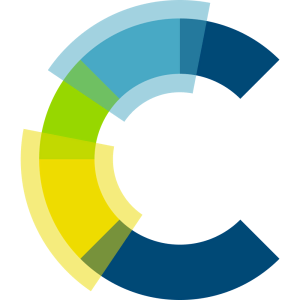
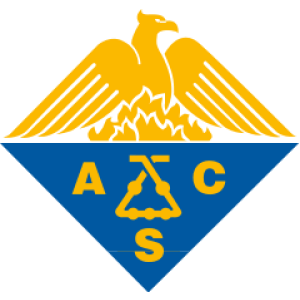
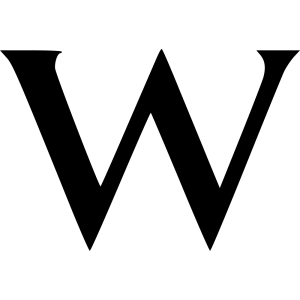
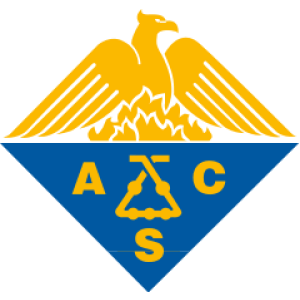
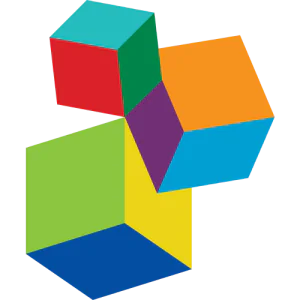
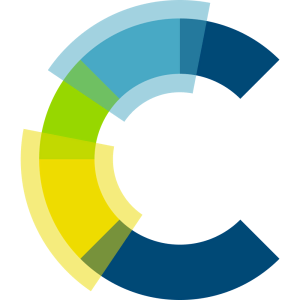
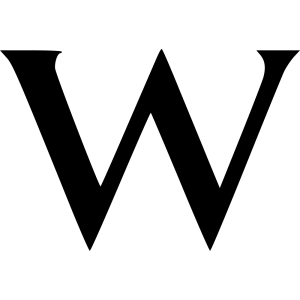
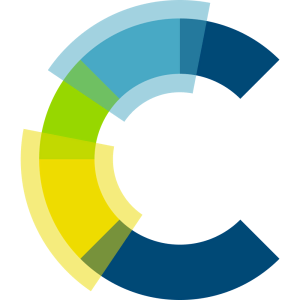
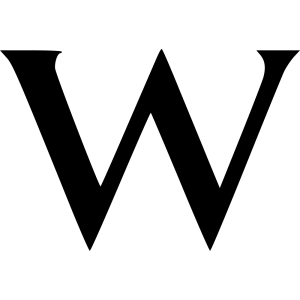
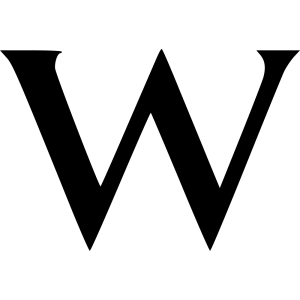
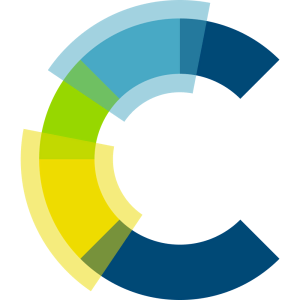
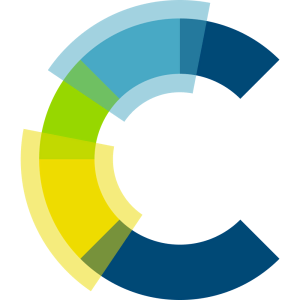
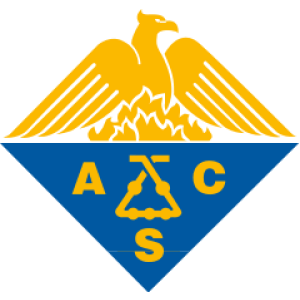
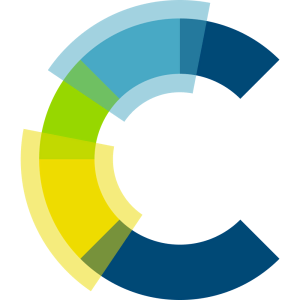
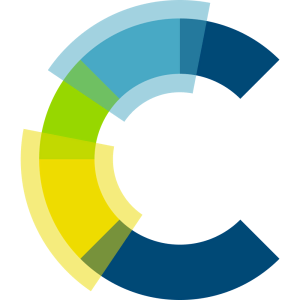
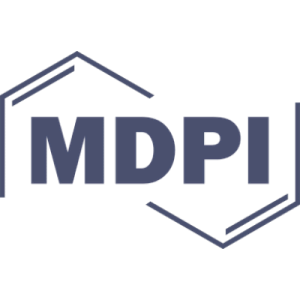
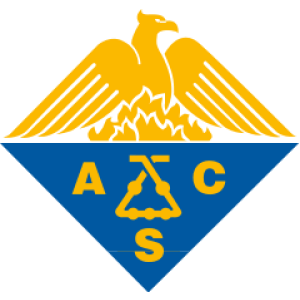
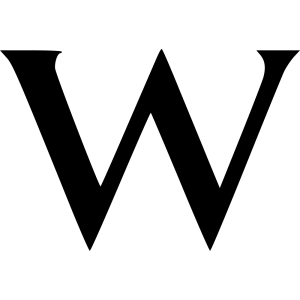
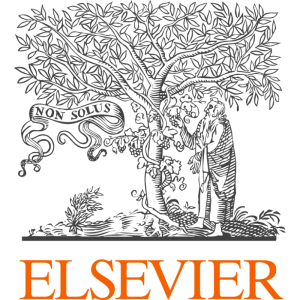
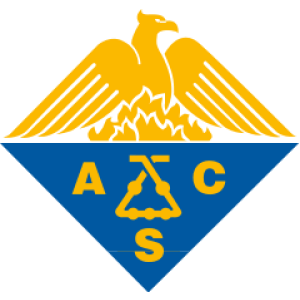
![Synthesis and characterization of oxygen-containing imidazo[1,2-d][1,2,4]oxadiazole fused-ring energetic compounds](/storage/images/resized/GDnYOu1UpMMfMMRV6Aqle4H0YLLsraeD9IP9qScG_small_thumb.webp)
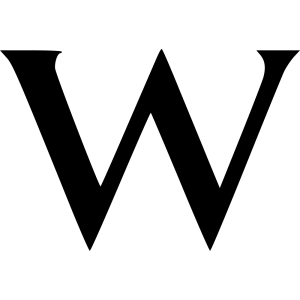
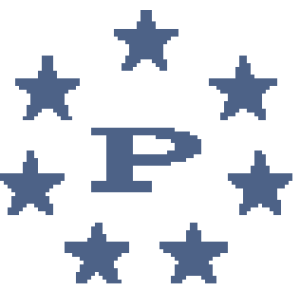
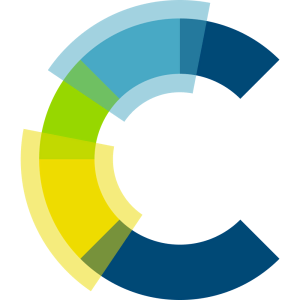
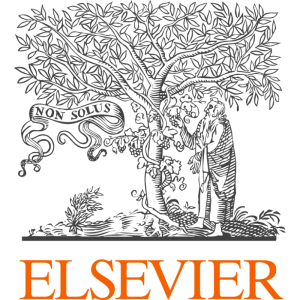
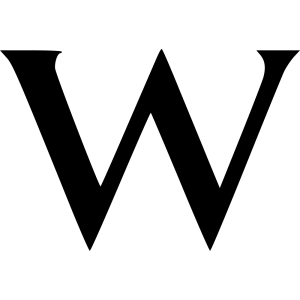
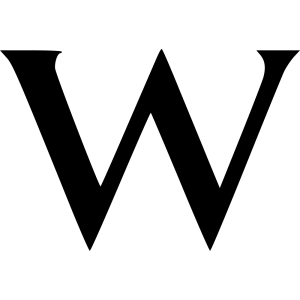
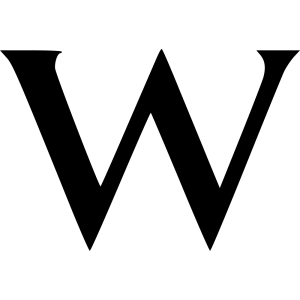
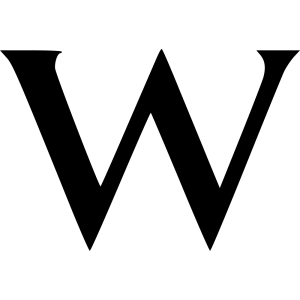
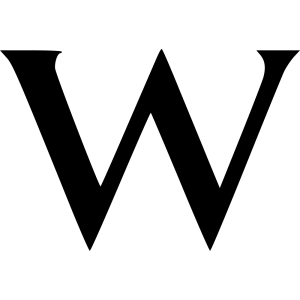
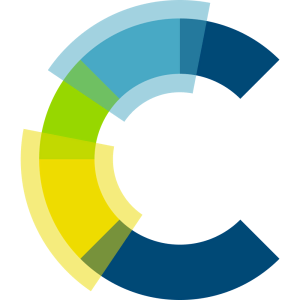
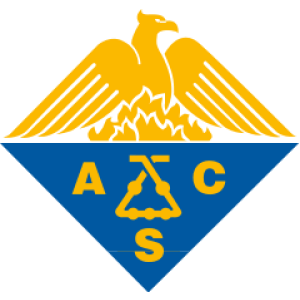
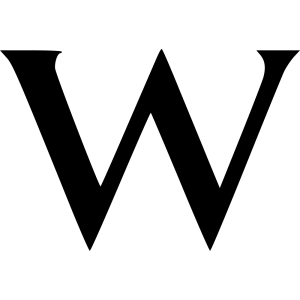
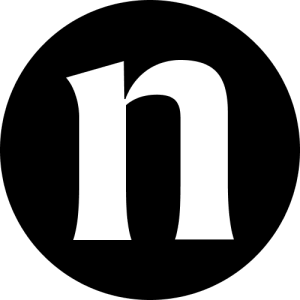
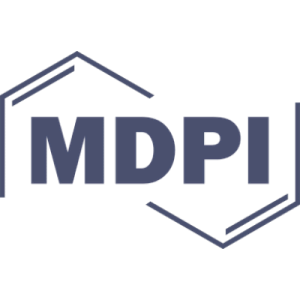
![Effect of counter-ion on packing and crystal density of 5,5′-(3,3′-bi[1,2,4-oxadiazole]-5,5′-diyl)bis(1H-tetrazol-1-olate) with five different cations](/storage/images/resized/xG3oC19920mmFVqkoVEGKGaMCeoalzyvWxPkVox5_small_thumb.webp)
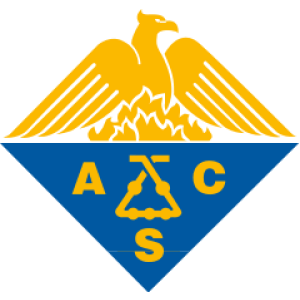
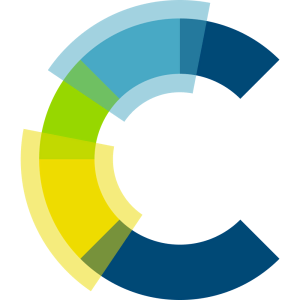
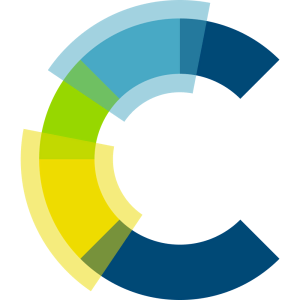
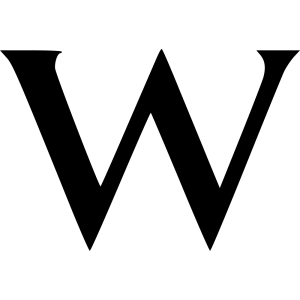