Keywords
Abstract
Rapid processes of tetrazole decomposition serve as sources of chemical energy stored in the five-membered ring, as well as gaseous products, primarily molecular nitrogen. Due to these properties, energetic tetrazole derivatives have found applications in various fields of science, engineering and technology, for example as components of energetic materials and products, as well as in emergency rescue equipment. The review presents an alternative view of the processes of tetrazoles decomposition, focusing on the diversity, differences, and similarities of the mechanisms and degradation products formed under the action of external energy sources. Some of these products are valuable reagents for the synthesis of previously inaccessible substances, as well as promising objects of analytical, medical, and bioorthogonal chemistry.
The bibliography includes 140 references.
1. Introduction
Tetrazole 1(N1H) and some of its derivatives were first synthesized by Bladin[1] in 1885 (see also anniversary article[2]). Herein, compounds bearing an unsubstituted nitrogen atom are indicated by NH next to the number in parentheses; for tautomers, the position of this moiety is given as the top index. Among the simplest stable azoles, this heterocycle has the highest nitrogen content and the highest enthalpy of formation (\( \Delta H_{f}^o \)) (Figure 1)[3, 4]. The unsubstituted pentazole (HN5), unlike its salts and N-aryl derivatives, has not yet been obtained in free form and has only been studied by computational methods[5].
Since the second half of the 20th century, the rapid processes of decomposition of tetrazole and its energetic derivatives, such as combustion and detonation, have been the subject of systematic studies by leading scientific schools, led by prominent scientists and specialists in the synthesis and chemical physics of energy-rich substances and materials. Tetrazole derivatives have received considerable attention in a number of monographs devoted to the chemistry, thermal decomposition, thermochemistry, combustion and detonation of energy-rich compounds and materials[6-10]. Below, in chronological order, are selectively cited original publications that reflect the important trends in the chemistry of energetic tetrazoles and the contribution of their authors to the development of this subject, which has not lost its relevance since the end of the XIX century and up to the present day[11-31].
The review articles and chapters of monographs[32-38] are devoted to the analysis and generalization of the findings in the chemistry and chemical physics of energetic tetrazoles, and also in the processes of tetrazole decomposition[32-38]. The topics of the traditional areas of chemistry and chemical physics of energetic tetrazoles related to thermal decomposition processes are still topical, as shown by the works published in recent years[31, 39, 40]. Figure 2 presents the exemplary formulas of typical energetic tetrazoles, with the name of the author and the year of preparation given below.
The chemistry of energetic tetrazoles, as evident from the above, goes back to the 19th century, but continues to develop intensively in the 21st century. The main trend is the search for novel compounds, the properties of which allow a compromise between the high energy amount released during rapid combustion or detonation and safe handling, which is determined by sensitivity to mechanical effects (impact, friction), fire beam, electrical discharge, coherent light sources and other primary impulses[40].
In addition to the traditional research area, a new, alternative vector of development in tetrazole chemistry, focused on the tetrazole ring initiated by external energy sources, has been intensively formed in recent decades. The great Rolf Huisgen (1920 – 2020) was at the origin of this scientific field[41]. This area has recently received additional impetus with the award of the Nobel Prize in Chemistry in 2022 to a group of scientists, Carolyn Bertozzi, K.Barry Sharpless, Morten Meldal, who have explored click and bioorthogonal chemistries. Obviously, it is practically impossible to use rapid thermal decomposition of energetic tetrazoles for synthesis and study of further product transformations. The risk of an uncontrolled transition from decomposition to detonation of such compounds should be considered. However, exceptions are known. For example, a successful synthesis of bis(1,2,4-triazolo[1,5-b;5',1'-f ])-1,2,4,5-tetrazine by thermocyclization of the energetic 3,6-bis(1H–1,2,3,4-tetrazol-5-ylimino)–1,2,4,5-tetrazine (BTATz) has been described[39]. Astakhov et al.[42] managed, although not without difficulty, to find conditions for studying the kinetics and mechanism of decomposition of the energetic 5-nitraminotetrazole. Nevertheless, tetrazole derivatives bearing no explosophoric substituents are undoubtedly more suitable models. It is known that the replacement of explosophoric groups on endocyclic nitrogen and carbon atoms, such as NO2, NHNO2, C(NO2)3, N3, N=N, etc. (see Figure 2), with aryl, certain hetaryl, alkyl, alkoxyl substituents in non-annulated heterocycles[38] makes these tetrazole derivatives not only safe to handle but also in demand as active ingredients of modern drugs[43]. Despite the still sporadic and scarce publications on this subject, studies of processes involving annulated tetrazole compounds and their degradation products[44, 45] are also of considerable interest in this context (Figure 3).
This review highlightes the findings on tetrazole decomposition where the authors have been able to detect, identify and in some cases isolate the decomposition products of non-annulated and annulated tetrazoles initiated by external energy sources. These processes occur without catalysts under the action of high temperature (thermolysis, pyrolysis), light (photolysis), radiation (radiolysis). A separate Section of this review is devoted to studies in which the fragmentation of the tetrazole ring occurs under mass spectrometric conditions.
2. Thermal decomposition of tetrazoles
The research findings on the thermal decomposition of unsubstituted tetrazole, its N- and C-derivatives, both non-annulated and annulated, and polyvinyl tetrazoles published before 1992 are summarized in the fundamental review by Gaponik and co-workers[33]. The data presented demonstrate the diversity of mechanisms, intermediates and products of thermal decomposition of tetrazoles, as well as the dependence of their rate and activation parameters on the nature of the substituents, temperature, solvent polarity and other factors. Special mention should be made of the pioneering work of Huisgen and co-workers[46-48] on the mechanisms and products of thermal decomposition of tetrazoles, cited in the review[33]. It was on the basis of these works that the idea of nitrile imines as key reagents (1,3-dipoles) for 1,3-dipolar cycloaddition was formed. Huisgen’s ideas enabled a systematic approach of a number of researchers to the interpretation of the results of quantitative studies of the above process.
The results of a manometric study of the kinetics of the thermal decomposition of 2,5-diaryltetrazoles in 1-chloro-naphthalene at 165.8 °C are described[49]. The reaction rate was monitored by the amount of nitrogen released. Based on the value of the parameter ρ of the correlation dependence of the logarithms of the observed first-order rate constants on the electronic constants of the Hammett substituents, as well as on the estimation of the activation entropy, the authors suggested that there is no symmetrical transition state of the retro–1,3-cycloaddition type in the mechanisms of the high temperature-induced nitrogen cycloelimination from 2,5-diaryltetrazole. First, the N(2) – N(3) bond is cleaved to generate an open-chain intermediate followed by elimination of the nitrogen molecule. Differential Scanning Calorimetry (DSC) has also been used to determine the kinetic and activation parameters of the thermal decomposition of tetrazoles[50, 51]. As the authors have shown, the activation parameters of tetrazole decomposition depend rather strongly on the conditions of the experiment, especially on the heating rate.
In later studies[52, 53], the kinetic and activation parameters of the thermal decomposition of 1,5-disubstituted (2a – e), 2,5-disubstituted (3a – e) and NH-tetrazoles 4a – h have been quantified (Scheme 1). The studies were carried out by the manometric method under isothermal conditions in Bourdon vessels in the gas phase (at 180 – 230 °C) and in solution in nitrobenzene (150 – 190 °C). It was shown that the thermal decomposition of 1,5-disubstituted tetrazoles 2a – e occurs predominantly via pathway 1 with the ring opening to afford azidoazomethine 5 (see Scheme 1). Release of N2 from the azidoazomethine 5 leads to nitrene 6, the subsequent sextet rearrangement of which gives carbodiimide 7. In the case of 2,5-disubstituted tetrazoles 3a – e, pathways 7 – 9 are realized, yielding compounds 8, but the preferred direction of thermal decomposition is pathway 9, leading to reactive nitrile imines 9, which often act as 1,3-dipoles in 1,3-dipolar cycloaddition reactions[47]. The unimolecular decomposition of the NH-unsubstituted tetrazoles 4a – h initially involves the rapid reversible interconversion of the 1H and 2H tautomeric forms, each of which decomposes by a specific mechanism. The 1H tautomeric form of such compounds is characterized by the reversible formation of azidoazomethine 5(NH) and further elimination of N2 to give nitrene 6(NH). The subsequent sextet rearrangement of this nitrene gives the corresponding carbodiimide 7(NH). The reversible ring opening in the tautomeric 2H-form of NH-unsubstituted tetrazoles leads in the limiting step to the diazo compound 8(NH) and in the next rapid step to its decomposition with loss of N2 and formation of the corresponding nitrile imine 9(NH) (see Scheme 1).
Analysis of the effect of substituents on the rate of thermal decomposition of tetrazoles indicates the crucial role of prototropic (N1H ↔ N2H) tautomerism as a factor affecting the mechanism of thermal decomposition of NH-unsubstituted tetrazoles[53] (see Scheme 1). The authors of an earlier study on the kinetics of thermal decomposition of 5-aryltetrazoles take a different view. In their opinion, the acid dissociation of NH-unsubstituted tetrazoles furnishing the corresponding anion, tetrazolide, contributes more significantly to this process[54]. The Section ‘Stability of tetrazoles’ of the well-known monograph[7] is based on the findings described in publications[52, 53] cited above. This monograph, which is well known to those skilled in the art of combustion and explosion, supports the conclusion of the authors of the study[53] who pointed out the determining influence of the prototropic tautomerism on the mechanism of thermal decomposition of NH-unsubstituted tetrazoles. The analysis of the activation parameters of tetrazole degradation presented in the above papers is of independent interest. However, given the diversity of tetrazole degradation mechanisms, it is practically impossible to summarize this problem within the scope of this review. The activation parameters of this process are analyzed in the monograph by Manelis et al.[7] and also in a series of articles by Kiselev and Sinditskii et al[55-57].
In recent years, an increasing number of papers have been devoted to the study of the thermal decomposition of tetrazoles using flash vacuum pyrolysis (FVP)[58]. Flash vacuum pyrolysis, despite very high operating temperatures, often reaching 600 °C and higher, is a promising method for the generation and control of decomposition products, including reactive intermediates capable of participating in secondary chemical and biochemical reactions, due to specific engineering solutions and hardware design[59]. FVP data allow us to significantly extend and deepen previously established ideas about the mechanism of thermal decomposition of tetrazoles. For example, it was shown[60] that nitrile imines[9], including those with R2 = H (see Scheme 1), can exist in three isoergic forms, namely, allenic, propargylic and carbenic (Scheme 2). Carbenic nitrile imines have maximum energy and are stabilized by electron-donating substituents. In addition to nitrile imines 9, 1H-diazirines 10, imidoyl nitrenes 6 and carbodiimides 7, including cyanamides 7(NH), are also involved in the general decomposition scheme (see Scheme 2)[60-62].
Flash vacuum pyrolysis of tetrazoles 4e,i is a convenient source of reactive compounds such as nitrile imines 9e,i, aryl diazo compounds 11e,i, N-nitrile imines 12e,i, indazoles 13e, cyanopyridine N-imides 14e,i. Theoretical studies using Density Functional Theory (DFT) at the CASPT2 level revealed the possibility of a transfer of the hydrogen atom from the pyrrole-type tetrazole 4i to the pyridine nitrogen atom with subsequent loss of N2 and formation of 1H-2-(diazomethylidene)pyridine 14i with an energy barrier of ~ 26 kcal mol–1 (Scheme 3)[62]. This simple pathway has not been considered before.
Study[59] presented the results of the research of NH-unsubstituted 5-phenyltetrazole (4e) under FVP conditions. The authors showed that in addition to the expected intermediates and products, benzonitrile and HN3 are also formed (see Scheme 3).
The decomposition of 1-(thiophen-2-yl)-1H-tetrazoles under microwave (MW) activation and FVP conditions has been studied[63]. The composition and yield of the thermolysis products depend strongly on the process conditions. Two examples from the extensive material of publication[63] are given below. Notably, despite the low yields, this work clearly demonstrates the possibility of synthesizing annulated pyrimidines and imidazoles as the main products of FVP. The thermolysis of ethyl 4,5-dimethyl-2-(1H-tetrazol-1-yl)-thiophene-3-carboxylate (15) was carried out at 165 °C in 1,2,4-trichlorobenzene (1,2,4-TCB) under microwave irradiation in the presence of benzylamine. As a result, 2-benzylamino-5,6-dimethylthieno[2,3-d]pyrimidin-4-ol (16) was obtained in moderate yield (Scheme 4)[63].
The FVP of methyl 2-(1H-tetrazol–1-yl)-4,5,6,7-tetrahydrobenzo[b]thienophen-3-carboxylate (17) gives methyl 5,6,7,8-tetrahydro–1H-benzo[4,5]thieno[2,3-d]imidazol-1-carboxylate (18) in low yield[63]. A possible mechanism for this reaction involves elimination of N2 from the tetrazole and further intramolecular attack of the terminal nitrogen of imidoyl nitrene on the carbon atom at the 3-position of the thiophene ring, followed by electrocyclization. The process is completed by the [1,5]-sigmatropic shift of the methoxycarbonyl group (Scheme 5).
The tetrazoles 1(N1H), 1(N2H), and the carbene 19 were the objects of theoretical calculations carried out using high-level ab initio quantum chemical methods[55]. The authors suggest that not only the tautomers of the unsubstituted tetrazole 1(N1H) and 1(N2H) (see Figure 1 and Scheme 1), but also the carbenoid structure 19 are involved in the thermal decomposition, the latter apparently being the most active (Scheme 6)[55]. This study presents the results of calculations of the energy parameters of the equilibrium constants (K1, K2) between different tautomeric forms of NH-unsubstituted tetrazole, the values of the rate constants k1, k2, k3 of monomolecular thermal decomposition, and also the parameters of the Arrhenius equation (Ea, logA) for the corresponding transformations.
The thermal decomposition of the prototropic tautomeric forms of 5-methyltetrazole 20(N1H) and 20(N2H) has been studied by experimental and theoretical methods[64]. The experiments involved the UV photoelectron spectroscopy monitoring of the thermal decomposition products of a sample of 5-methyltetrazole subjected to rapid and slow (< 60 °C per min) heating from room temperature to 500 °C. The theoretical study was carried out using data from quantum chemical calculations by the MP2/6-311++G(d,p) method and taking into account the earlier concepts[55] (see Scheme 6). It was found that the thermal decomposition of the tautomer 20(N2H) prevailing in the equilibrium mixture starts in the gas phase at 195 °C and gives low-molecular-weight compounds, N2, MeCN and HCN (Scheme 7).
The nitrogen molecule is formed by two competing pathways from the 5-methyltetrazole tautomers 20(N2H) and 20(N1H) with activation barriers to isomerization of 150.2 and 126.2 kJ mol–1, respectively. Two competing pathways with activation energies of 218.3 kJ mol–1 from the 20(N2H) tautomer and 198.6 kJ mol–1 from the 20(N1H) tautomer also lead to acetonitrile. According to quantum chemical calculations, the formation of HCN results from the secondary reactions with thermal decomposition products, MeCN and MeN=C=NH[64].
The problem of activation of thermal decomposition of tetrazoles has been considered on the example of 2,5-dimethyltetrazole (21), 2-methyl-5-phenyltetrazole (22), 5-methyl-2-phenyltetrazole (23) and 2,5-diphenyltetrazole (24), as well as other substituted tetrazoles.65 The data obtained using the M06-2x functional and the 6-31+G(d,p) level of theory with the solvation model (SMD) corrections, indirectly indicate the possibility of a significant (~ 80 kJ mol–1) lowering of the activation barrier for the thermal decomposition of tetrazoles using the most suitable solvents and introducing an electron-withdrawing moiety in the substituent at the N(2) tetrazole atom[65].
The thermal decomposition of 5-aminotetrazole (25) has received close attention[4]. This nitrogen-rich representative of the azoles has a high enthalpy of formation but is safe to handle[4, 6]. Both tautomeric forms 25(N1H), 25(N2H), and 1,4-dihydro-5H-tetrazol-5-imine (26) may be involved in its thermal decomposition (Figure 4).
As noted by Paletsky et al.[66] with reference to studies[67, 68], the mechanism of thermal decomposition of 5-aminotetrazole implies the involvement of both prototropic tautomeric aminoforms 25(N1H) and 25(N2H), and the isomeric imino-form 26, which converts to the tautomer 25(N1H) on melting. The above forms give rise to gaseous (HN3, N2, NH2CN, NH3, HCN) and condensed (melamine 27) products. Hydrazoic acid, in turn, decomposes with the release of energy which intensifies the decomposition of the starting sample of 5-aminotetrazole (25). Such a process can be achieved by self-sustained combustion of compound 25 under pressurised conditions (Scheme 8)[66].
A generalized mechanism for the thermal decomposition of 5-aminotetrazole, which complements the results of studies[66, 67, 69], is reviewed[59] (Scheme 9).
The results of a theoretical study (DFT B3LYP technique) of the thermal decomposition of 5-aminotetrazole 25 in the gas phase and in the melt (simplified model) are reported[70]. As can be seen from the analysis of the energy diagrams and the values of the activation parameters, the thermolysis of the imino form 26 in the condensed phase (melt) cannot be described only by the traditional concept of the unimolecular mechanism. The formation of intermediate reactive dimers should be considered. The dimers 28 and 28' can be formed with the participation of hydrogen atoms from both the NH2 group of compound 28 and the endocyclic NH group of tetrazole 28'. In the latter case, the hydrogen bond is formed with a pyrrole-type nitrogen atom (Figure 5).
The thermolysis of 5-aminotetrazole (25), 5-amino-1-methyltetrazole (29a), 1,5-diaminotetrazole (30) and the sodium salt of 5-aminotetrazole 31 was studied using a set of complementary experimental methods: thermogravimetric analysis (DSC, DTA), thermal volumetric analysis (TVA), evolved gas analysis (EGA), infrared spectroscopy, mass spectrometry, and gas chromatography[67, 68]. Data from kinetic experiments are also included in the discussion. For 5-aminotetrazole (25), two main routes of thermal decomposition, determined by the involvement of amino and imino forms, were confirmed. Thus, heating of compound 25 increases the content of imino form 26, the melting and decomposition of which occur simultaneously to give hydrogen azide and carbodiimide. The polymerization products of carbodimiimide and melamine 27 were detected in the condensed phase. Subsequent heating leads to the decomposition of the corresponding amino form 25(N1H), accompanied by nitrogen release. It is shown that secondary reactions allow the range of products of thermal decomposition of 5-aminotetrazoles to be significantly extended[67, 68]. It should be stressed again that the above experimental data[67, 68] are consistent with the mechanism presented in the study[66].
The thermal decomposition of 1-(R-phenyl)tetrazoles, where R = H (32), 2-Cl (33), 4-Cl (34), 4-OH (35), 4-OMe (36), 4-NO2 (37), 1-(3-pyridyl)tetrazole 38 and 1,4-bis(tetrazol–1-yl) benzene (39) has been studied by theoretical (B3LYP/cc-pVDZ level of theory) and experimental methods (TGA, DSC and IR spectroscopy)[69]. The ortho- and para-substituted 1-aryltetrazoles 33, 34 (R = Cl) and 36 (R = 4-OMe) have been shown to decompose at 190 – 240 °C with energy release and formation of the corresponding aryl isonitriles and a binary mixture of gaseous products consisting of 1.5 mol of N2 and 0.5 mol of H2. The regularities of thermal decomposition of 1-phenyltetrazole (32) and its 4-nitro derivative 37 differ from those mentioned above. According to the authors, this is due to the different nature of the electron density delocalization. For example, in the case of compound 37 (R = NO2), the nitro group is reduced during decomposition. The presence of two tetrazole units in 1,4-bis(tetrazol–1-yl)benzene (38) leads to a loss of 95% of the molecular mass during decomposition[71].
The chemistry of tetrazole-containing polymers and materials based thereon has traditionally attracted the attention of researchers[35]. As an example, we present the results of the decomposition of the tetrazole ring in polyvinyltetrazoles. The thermal decomposition of poly(1-vinyl-5-methyltetrazole) 39 and poly(1-vinyl-5-phenyltetrazole) 40 has been studied using high-resolution thermogravimetry, DSC and thermometry methods[72]. As the temperature increases, the polymers 38 and 39 decompose stepwise and the process begins, as expected, with the degradation of the tetrazolyl moieties. Subsequent transformations are due to the formation of active intermediates such as azides 41, imidoyl nitrenes 42, as well as products of subsequent transformations of macromolecules 43 and 44, which takes place in the temperature range 210 – 290 °C. Polymer chain degradation occurs at temperatures ≥320 °C (Figure 6)[72].
In the case of annulated tetrazoles, the azido-tetrazole equilibrium is of constant interest[44, 45]. However, the works devoted to the study of the thermal decomposition patterns of annulated tetrazoles and to the determination of the nature of the resulting products are represented by single examples. For example, as shown in publication[73], FVP of annulated benzene rings of hexa- and heptamethylenetetrazoles (45, 46) furnishes 9- and 10-membered cyclic carbodiimides 49, 50 respectively, instead of the expected cyanamides 47, 48 (Scheme 10).
To conclude the Section on the thermal decomposition of tetrazoles, it should be noted that the development of this interesting and promising subject would not be possible today without the pioneering studies in the field of the straightforward synthesis and chemical physics of energetic tetrazoles carried out at the end of the last century by scientists and specialists of specialized research institutes, design bureaus and university teams of the USSR, whose references are given in the Introduction. With the development of new methodologies for decomposition processes such as FVP, where their products can be synthesized and controlled, new results on thermal decomposition studies of both non-annulated and annulated tetrazoles can be expected in the near future.
3. Photodegradation of tetrazoles
Tetrazoles are extremely interesting and promising objects of photochemistry. Researchers are attracted by the possibility of inducing controlled degradation of the tetrazole ring under the action of light of a certain wavelength, including coherent radiation generated by lasers[74, 75]. The conditions of formation of products of photochemical decomposition of tetrazoles are much milder compared to thermal decomposition, which facilitates analytical control of intermediate and final products. Moreover, these products can be used as reagents in further chemical and biochemical reactions for the synthesis of practically relevant compounds of a given structure. There are several methods of photochemical decomposition currently being developed by leading laboratories around the world, which are briefly described in this Section.
3.1. Photolysis of tetrazoles in cryogenic matrices
The review[75], with reference to the original publication[76], presents a scheme of photoinduced decomposition of unsubstituted tetrazole 1(N2H) in a cryogenic matrix (deposited in solid argon at 12 K). Photolysis under the action of a 193 nm coherent light source (ArF laser) leads to photodegradation of the tetrazole, accompanied by elimination of the N2 molecule and formation of a diversity of photochemical transformation products, including nitrile imines, HCN – NH complex, diazomethane, carbodiimide and cyanamide (Scheme 11).
The structure of nitrile imines generated by photoinduced degradation of 2,5-diaryltetrazoles in cryogenic matrices is reviewed in article[77].
Spectral methods such as Fourier-transform infrared spectroscopy (FTIR) have been successfully used to monitor the products of chemical transformations occurring during the photoinduced decomposition of tetrazole derivatives in isolated cryogenic matrices. Using this method for tetrazoles 29, 51a – c, 52a – c, it was possible to determine the patterns of photoinduced fragmentation of the tetrazole ring up to the identification of the individual bond cleavage with fixation of the corresponding products in the matrix (Figure 7)[78, 79].
The effect of substituents on the process of photoinduced decomposition of some tetrazoles has been studied in cryogenic matrices, which is a harsh environment of solidified noble gases (argon, xenon) at temperatures of 10 K. Under these conditions, the products are formed and stored in a fixed state, directly in the matrix cell, without being involved in subsequent reactions. This greatly simplifies the sequence of transformations and allows to determine the structure of intermediates and some products by FTIR. A useful analytical tool is the ability to compare data from experimental and theoretical spectra calculated for the particle state in vacuo[79]. The results of such studies have been demonstrated for a number of 1,5-disubstituted tetrazoles. Scheme 12 and Scheme 13 illustrate, as examples, the photoinduced (λ > 235 nm) decomposition of 5-methoxy-1-phenyl-1H-tetrazole (52a) and 1-phenyltetrazolone (51a), respectively, in a cryogenic matrix.
As evident from Scheme 12, secondary transformation products of different structures were found in the case of compound 52a: 3-methoxy-1-phenyl–1H-diazirine (53), methyl isocyanate (54), phenyl azide (55) and 1-azacyclohepta-1,2,4,6-tetraene (56).
The result of the photochemical transformation of tetrazolone 51a includes several unexpected products. The authors of the cited publication[78] reported the formation of two conformers (with cis and trans configurations) of 1-phenyldiaziridin-3-one (57), phenyl isocyanate (58), the already mentioned 1-aza-cyclohepta–1,2,4,6-tetraene (56), isocyanic acid (59), 3-phenyl-2,3-dihydrotetrazete (60), as well as cis and trans isomers of phenyldiazene 61 (see Scheme 13).
The photoinduced decomposition of tetrazoles in cryogenic matrices is of considerable practical interest as a tool for monitoring the stability of active pharmaceutical ingredients (APIs) of drugs to electromagnetic radiation in the ultraviolet and visible regions of the spectrum. An example of such a method is given in a publication[80], which is devoted to the study of N-methyltetrazolylsaccharinates. Such compounds have attracted attention as catalysts for the oxidation of primary and secondary alcohols and as promising drug candidates with strong cytostatic properties[80]. The authors present the routes of photoinduced decomposition of regioisomeric N-methyl-tetrazolylsaccharinates exposed to narrowband UV irradiation at different wavelengths. The process was monitored by infrared spectroscopy with ab initio quantum chemical calculations used to interpret the data. The present work was preceded by research by the same authors on the photoinduced decomposition of regioisomeric 5-amino–1-methyl- and 5-amino-2-methyl-tetrazoles 29a,b in cryogenic argon matrices at 15 K. Simple arrows indicate photolysis routes of tetrazoles 29a,b upon irradiation with light of wavelength λ = 222 nm and λ = 250 nm, respectively. Dashed arrows indicate photolysis routes upon irradiation with light of wavelength λ = 325 nm (Scheme 14).
When comparing the results of the photochemical decomposition of regioisomeric N-methyltetrazolylsaccharinates and regioisomeric N-methyl-5-aminotetrazoles 29a,b (see Scheme 14), general directions of photoinduced decomposition were identified. For example, the possibility of the formation of 1-methyl–1H-diazirin-3-amine (62), a common intermediate for isomers 29a,b, has been demonstrated. It was notes that functionalization of the 5-amino group of the tetrazole ring has a significant stabilizing effect[81]. According to the authors, this effect could be useful in the design of model compounds specifically intended to explore the mechanism of tetrazole decomposition in cryogenic matrices.
The photoinitiated degradation of 5-methyltetrazole 20 was studied in a solid cryogenic argon matrix at 10 K. A tunable UV laser system was used to study photodegradation processes at different wavelengths. The reactions were monitored by FTIR spectroscopy. According to the vibrational spectra, the 5-methyltetrazole isomer 20(N2H) predominated in the cryogenic matrix. The results were interpreted using kinetic methods and quantum chemical calculations (DFT and its time-dependent variant (TD-DFT)). As a result, three products of the tetrazole ring degradation were successfully identified such as C-methylnitrile imine (63), N-methylcarbodiimide (64) and methyl cyanamide (65). In turn, N-methylcarbodiimide 64 further decomposes into two molecules of HCN or HNC. The latter are in equilibrium in the matrix cells and further form various dimers through hydrogen bonding (Scheme 15)[82].
Photolysis of 2-(tetrazol-1-yl)- (66), 3-(tetrazol-1-yl)- (67) and 2-(tetrazol-5-yl)pyridines (68) in cryogenic argon matrices leads to 2-pyridyl- (69) or 3-pyridylcarbodiimide (70)[83]. The formation of a unique product of the rearrangement occurring during photolysis, 1-cyclopenta-2,4-dienylketenimine (71), has also been detected by spectral analysis. The formation of ketenimine (71) could be explained by using quantum chemical calculation (B3LYP/6-311þþþG(2d,2p)) data (Figure 8).
Despite the unique possibilities and prospects of using cryogenic matrices for the elucidation of structure of tetrazole photolysis products, it should be noted that the isolation of such products in pure form, e.g. by extraction methods, is a challenging task. This limits the use of photoinduced degradation of tetrazoles as an effective method for the directed synthesis of organic compounds[75, 84].
According to the authors of the above-cited papers, the photodegradation of tetrazoles in organic solvents is a more promising strategy with applications in the synthesis of new or otherwise difficult to obtain compounds.
3.2. Photolysis of tetrazoles in organic solvents
The use of different organic solvents and varying the conditions of the photochemical processes can affect the selectivity and the yield of the target photoproducts[75]. This Section reviews publications dealing with the synthesis of individual compounds by photodegradation of tetrazoles in solution.
Hyatt и Swenton[85] pioneered the photochemical synthesis of 9H-pyrimido[4,5-b]indoles 72 from annulated 8-(para-substituted)tetrazolo[1,5-c]pyrimidines in trifluoroacetic acid using a 16 RPR-3000 Å lamp. Quast and Bieber[86] described methods for the synthesis and results of a photolysis study of 1,4-dialkyl–1,4-dihydro-5H-tetrazol-5-ones and -5-thiones. Later, Quast and Nahr[87] reported the synthesis of alkenyldiaziridinones 73a – c by photochemical degradation (λ = 254 nm, 20 °C, degassed sample at 10 – 5 Torr, 150 W Hg lamp) of the corresponding 1-alkenyl-4-tert-butyl–1,4-dihydro-5H-tetrazol-5-ones. The same authors[88] showed that 1-allyl-4-alkyltetrazol-5-thiones being irradiated (λ ≥ 254 nm, high pressure Hg lamp, 20 °C) in methylcyclohexane-d14 and acetonitrile-d3 furnish the corresponding carbodiimides 74 in 50 – 80% yields. The synthesis of benzimidazolones 75 in near quantitative yields by photolysis of 1-phenyl–1,4-dihydro-5H-tetrazol-5-ones (λ = 254 nm, 150 W Hg lamp, 20ºC) in methanol, acetonitrile or propan-2-ol was also reported[88]. In a follow-up publication[89], the preparation of two classes of compounds, hexahydronaphthyridines 76 (λ ≥ 320 nm, high pressure Hg lamp, −60 °C, 1.25 h, 95% yield) and iminoaziridines 77 (λ ≥ 305 or 320 nm, high pressure Hg lamp, 30 – 50% yield) by photolysis of 5-alkylidene-4,5-dihydro–1H-tetrazoles in toluene-d8 was presented. The successful synthesis of 2-phenylamino–1,3-oxazines 78 by photolysis of 5-allyloxy–1-aryltetrazoles carried out under low pressure mercury lamp irradiation (λ = 254 nm) in methanol, acetonitrile and cyclohexane was published (Figure 9)[75, 90].
According to the authors of the review[75] published in 2010, the photolysis of tetrazoles in organic solvents is one of the most attractive strategies for the synthesis of various products. The selectivity of such photochemical processes can theoretically be controlled and modified towards the formation of desired products by choosing solvents and reaction conditions. At the same time, a common feature of the photochemical degradation of tetrazoles in organic solvents is the release of molecular nitrogen, whereas in cryogenic matrices, many uncontrollable alternative patterns of the tetrazole ring cleavage are realized.
In some cases, the effect of the solvent on photodegradation is particularly pronouced, but it is sometimes difficult to predict the nature of the resulting products. For example, unexpected results were obtained by nanosecond laser photolysis of 5-benzoyl-1-methyltetrazole (79). Photolysis in H-donor solvent (propan-2-ol), apolar solvents (heptane, cyclohexane) and finally in aprotic dipolar solvent (acetonitrile) gave various products 80 – 83 (Scheme 16)[91].
3.3. ‘Photoclick’ chemistry
The last decade has seen a cardinal revaluation of the role of photodecomposition of tetrazoles as an approach to new or hard-to-reach organic compounds of practical value. New strategies of so-called ‘photoclick’ chemistry are oriented towards the use of active intermediates formed in situ during the photoinduced decomposition of the tetrazole ring in reactions with externally introduced compounds, mostly nucleophilic agents. It is worth recalling that pioneering work in this field of tetrazole chemistry was published in the late 1950s by Huisgen et al[47, 92, 93]. (see also anniversary article [41]). The photoclick chemistry strategy has gained prominence in modern science since the award of the 2022 Nobel Prize in Chemistry (see Introduction). The 1,3-dipolar cycloaddition of azides to nitriles leading to the formation of the tetrazole ring, as well as reactions involving active intermediates formed by its decomposition, are among the limited number of transformations that are in line with the concept of bioorthogonal chemistry first formulated by Bertozzi[94]. These transformations include the photo-decomposition of 2,5-diaryltetrazoles 84 to give intermediate nitrile imines 85, dipoles which undergo a cycloaddition with alkenes 86 as dipolarophiles to furnish the corresponding 1,3-diaryl derivatives of 4,5-dihydro–1H-pyrazoles 87 (Scheme 17)[95].
It should be noted that the dynamic development of this concept is directly related to the successes in the elaboration of rational and selective methods for the synthesis of 2,5-diaryl- (84) and 2,5-dihetaryltetrazoles as the most promising starting materials required in the ‘photoclick’ chemistry methodology[96].
Figure 10 shows examples of 2,5-diaryltetrazoles (85 – 87) used in this methodology[97].
Since 2008, the trend in bioorthogonal chemistry based on photoinduced decomposition of 2,5-diaryltetrazoles and subsequent 1,3-dipolar cycloaddition involving nitrile imines and alkenes has become a priority, along with azide – alkyne and azide – nitrile variants. Noting the advantages and prospects of this strategy of photochemical synthesis, Sinha et al.[98] emphasize the need for an in-depth understanding of the mechanisms of chemical, physical and physicochemical processes associated with the photoclick chemistry. For its successful implementation according to the principles of bioorthogonal chemistry, one need information on the chemical structure and reactivity of the above-mentioned active intermediates generated during the photoinduced degradation of the tetrazole ring. The pathways for the formation and further transformation of intermediates such as nitrile imines, imidoyl nitrenes, etc. were previously mostly simply postulated[59], and quantum chemical methods did not always unambiguously determine their most stable form[99]. However, this situation has changed rapidly in the last 15 years. In 2020, a monograph[61] was published on the structure, properties and reactivity of nitrile imines. It systematized and discussed in detail the methods and mechanisms of formation of nitrile imines from 2,5-diaryltetrazoles[84] and cited the works in which the formation of nitrile imines was detected experimentally using spectral methodssuch as UV, IR and NMR spectroscopy.
Wentrup and co-workers[100] experimentally detected the formation of nitrenes 88 and 89 during the photolysis of 1-methyl-5-phenyltetrazole (2c) using EPR spectroscopy. In this case, photolysis gave methyl(phenyl)carbodiimide 90 as the final product (Scheme 18).
The monograph[61] paid considerable attention to the results of the study of the processes of 1,3-dipolar cycloaddition of nitrile imines 9, generated during the photolysis of 2,5-diaryltetrazoles 84, to various nucleophilic reagents. Lin and co-workers[101] rationalized the application of photoclick reactions involving inactivated alkenes (dipolarophiles) and nitrile imines (1,3-dipoles), which are products of the photoinduced decomposition of 2,5-diaryltetrazoles 84, to bioorthogonal chemistry. The 1,3-dipolar cycloaddition of 1,3-dipoles to the corresponding dipolarophiles leads to fluorescent pyrazoline cycloadducts, which can be used to control the process of protein functionalization in cells[101]. For example, a simple alkene tag, homoallylglycine (HAG), was cotranslationally incorporated into the recombinant protein as well as into endogenous, newly synthesized proteins in mammalian cells via 1,3-dipolar cycloaddition involving the terminal double bond of HAG and nitrile imines generated by photolysis of 2,5-diaryltetrazoles 84 (Scheme 19)[102].
Based on the findings of the authors of the above-mentioned papers, it can be concluded that tetrazole photochemistry is becoming a useful tool for priority research in modern medicine and biotechnology. For example, such chemical transformations may be in demand for photoradiochemical labelling of monoclonal antibodies (mAbs). The use of photoclick chemistry involving 2,5-diaryltetrazoles 84 in the synthesis of radiopharmaceuticals for positron emission tomography (PET) is reported[103].
Another interesting area of photoclick chemistry is the possibility of forming self-assembled microlayers (SAMs) in photoinduced microcontact printing[104].
However, as mentioned above, no universal methodology has yet been developed that would allow these processes to be used with high efficiency in bioorthogonal chemistry. Researchers are sometimes surprised by the structure and dual reactivity of active intermediates, such as nitrile imines 85[61]. Li et al.[105] stated that the photoinduced decomposition of 2,5-diaryltetrazoles 84 in the presence of nucleophilic reagents such as alkenes 86 does not always meet the requirements of bioorthogonal chemistry. For example, the photoinduced decomposition of 2-(4-methoxyphenyl)-5-phenyl-2H-tetrazole (91) in the presence of pent-4-enoic acid with two reaction centres furnished an unexpected product instead of the cycloaddition product of nitrile imine 92 on the terminal double bond of 3-[1-(4-methoxyphenyl)-3-phenyl-4,5-dihydro-1H-pyrazol-5-yl]propanoic acid (93). The nucleophilic attack of the carboxylate anion of pent-4-enoic acid on the carbon atom of the nitrile imine moiety of the protonated nitrile imine 94 gives benzhydrazone 95, which undergoes subsequent rearrangement to afford N-(4-methoxyphenyl)-N-(pent-4-enoyl)benzhydrazide 96 in 71% yield (Scheme 20)[105].
An important step in the implementation of the photoclick chemistry strategy is a choice of nucleophilic reagents for in situ reaction with nitrile imines generated during the photolysis of tetrazoles. In this respect, the publication[106], which focuses on the reactions of nitrile imine 93, generated during the photolysis of 2,5-diaryltetrazole 91, with nucleophiles 97a – f to give hydrazones 98 (Scheme 21), is of particular interest.
A combination of several spectral methods together with quantum chemical calculations is used to identify and determine the structure of nitrile imines. Attempts have also been made to introduce trap compounds into the reaction mixture that are capable of trapping active intermediates[107]. Another very important aspect of the methodology for studying the photoinduced decomposition of tetrazoles, namely, the dependence of the process direction on the wavelength of the excitation radiation, is outlined. The authors draw attention to the need for a quantitative study of the reactivity and mechanism of photoinduced decomposition of tetrazoles. Using a complex wavelength-tunable laser system to initiate photochemical processes, they managed to determine a number of quantitative patterns. This study was exemplified by the photoinduced decomposition in the presence of N-ethylmaleimide (NEM) of methyl 4-(2-phenyl-2H-tetrazol-5-yl)benzoate (99a) and polyethylene glycol (PEG)-based macromolecule 99b bearing a terminal 2,5-diphenyltetrazole moiety in the chain. As a result, derivatives of 5-ethyldihydropyrrolo[3,4-c]pyrazol-4,6(1H,5H)-dione 100a,b were obtained (Scheme 22)[107, 108].
3.4. Photolysis of 4,5-dihydro-1H-tetrazole derivatives
2,5-Diaryltetrazoles 84, the molecules of which have a π-electron system with a high aromaticity index, are mainly used as models to study the photoinduced decomposition of tetrazoles. As discussed above (see Scheme 17), the photolysis of 2,5-diaryl-tetrazoles 84 involves the losee of the N2 molecule to give reactive nitrile imines[61, 98, 109]. When the aromaticity of the cycle is disturbed, for example in the case of 4,5-dihydro-1H-tetrazoles, the transformation pathways can be very different. In the review[110], concerning the chemistry of 5-alkyl(aryl)- and 5-alkylidene-substituted 4,5-dihydro-1H-tetrazoles, attention is drawn to the study[111, 112] on the photolysis of such compounds. Based on these data, it can be confidently stated that both photolysis in an organic solvent (CH2Cl2) or cryogenic matrix and thermolysis of 1-methyl-4,5-dihydro-1H-tetrazoles 101 lead to the elimination of the nitrogen molecule and the formation of two enantiomeric diaziridines 102 and 103, the ratio of which is governed by the process conditions and the nature of the substituents R1, R2 and R3 (Scheme 23).
4. Fragmentation of tetrazoles under mass spectrometric conditions
Studies of tetrazoles by mass spectrometry began in the 1960s[113, 114]. The relationship between fragmentation products and the structure of molecular ions derived from regioisomeric 1-methyl- (2) and 2-methyl-5-aryltetrazoles (3) as a result of ‘hard’ electron impact ionization (70 eV) has been found[115]. Under electron impact, 1-methyl-5-aryltetrazoles 2 generates a molecular ion, which can exist in both cyclic (tetrazole) and open (azidoazomethine) forms due to the ring chain equilibrium[115]. It is the molecular ion 5+• in the form of azidoazomethine, which in this case is the main source of the products formed in the subsequent stages of fragmentation (Scheme 24).
The molecular ion produced through electron impact from 2,5-disubstituted tetrazoles 3 undergoes synchronous cleavage of the N(2) – N(3) and N(4) – C(5) bonds. This process determines both the effects common to the regioisomeric molecular ion 2+•, such as the loss of the N2 molecule, and the peculiarities of its further fragmentation. The recordred mass spectra show a preferential elimination of N2 , HN3 and HCN species[115]. Both the cited experimental study[115] and the subsequent review article[116] compare and summarize the results of independent studies of thermal decomposition (see Scheme 1) and mass spectrometric fragmentation of regioisomeric tetrazoles (see Scheme 24). The authors of both papers point out certain analogies in the decomposition patterns of tetrazoles initiated by different energy sources.
A characteristic trend in modern mass spectrometric studies of tetrazoles is the use of ‘soft’ ionization methods (e.g. electrospray). This is used, for example, to study the properties of the active pharmaceutical ingredients of modern tetrazole-containing drugs. These are usually multi-atomic systems with bulky substituents in the tetrazole ring. For example, soft ionization mass spectrometry is used to monitor the degradation pathways of the molecules of the active ingredients of sartans — modern drugs for the treatment of arterial hypertension[117-121]. In recent years, the use of these methods has become an essential requirement for the manufacture and storage of medicines under GMP regulations[119].
In addition to applied studies, soft ionization methods have recently been used to determine the individual molecular ion features of previously inaccessible tetrazole-containing compounds, e.g. for NH-unsubstituted 5-vinyltetrazole (104) (Figure 11)[118, 120-123]. Mass spectra often show molecular peaks of adducts with sodium or potassium, ions which are always present in trace amounts in the mobile phase[121, 123]. In the full mass range scan mode in the spectrum of tetrazole compounds, fragment ions generated via soft ionization are also detected. For example, the maximum intensity in the spectrum of 3-(5-phenyl-2H-tetrazol-2-yl)pyridine 105 in this mode is observed for the ion corresponding to the [M + H – N2]+ fragment, while the intensity of the protonated molecular ion is only 10%[96]. When recording fragment spectra of tetrazole-containing compounds, a characteristic elimination of the N2 molecule is observed[118, 120]. Other typical fragmentation pathways for relatively small molecules of tetrazole-containing compounds are also observed, with release of HCN and HN3 fragments. For example, such a result was observed when studying the fragmentation of 5-(1-aryl-1H-pyrrol-2-yl)-1H-tetrazoles 106a – g in positive and negative ion modes (see Figure 11)[118, 123].
To conclude this Section, it should be noted that both hard (see Scheme 24) and soft ionization methods show that fragmentation follows the specific patterns related to the structure of tetrazoles. Indirectly, this points to the major contribution of the nitrogen-rich tetrazole ring as a conservative factor determining the degradation pathways and the nature of the resulting species to the mechanism of mass spectral fragmentation during degradation.
5. Radiation destruction of tetrazoles
Studies of the effects of radioactive radiation on tetrazole-containing organic compounds are important not only from a scientific point of view, but also for solving practical problems. One such task is to determine the conditions for the storage and use of tetrazole-containing vital drugs and energetic compositions under increased radiation background in emergency situations and in space, as well as in the treatment and diagnosis of diseases using radiopharmaceuticals. For a number of compounds, it is the tetrazole ring, as the most energetic unit, that is capable of activating destructive processes. Some tetrazole derivatives can be considered as effective radioprotectors, trapping ionizing radiation and protecting more complex biological molecules from its impact[124].
The published data are mostly devoted to the evaluation of the effect of γ-radiation on the tetrazole ring. It should be noted that the classical systematic works in this field were carried out in the last century and have not been fundamentally revised. Berk and Gisser[125] pioneered the study of the γ-radiolysis of unsubstituted tetrazole 1 in 1973. It was shown that irradiation of tetrazole with doses ranging from 1.1 × 1020 to 4.7 × 1022 eV g–1 at 100 °C produced a large number of gaseous products (97% of the total), with this gas mixture consisting of 99% nitrogen. Destruction of the tetrazole ring was also observed at low irradiation dose (1.0 × 1018 eV g–1) and lower temperature with generation of radical species, as confirmed by EPR. The decomposition of the energetic 5-aminotetrazole (25) and its derivatives 107 – 111 on exposure to γ-irradiation has been discussed in several publications (Figure 12)[125-128].
Moorthy and co-workers 126 reported the results of experiments on the radiolysis of tetrazole 25 in aqueous solutions by high-energy electron pulses (7 MeV with pulse durations of 2 μs and 50 ns) obtained using a linear accelerator. The irradiation dose to the samples was 15 – 25 Gy/pulse. Experiments were carried out at pH 4.5 and 7.5, where 5-aminotetrazole is present in the neutral 25(N1H) and anionic 25(N-)forms. The secondary processes occurring in these solutions were also studied. These findings show that both forms eliminated ammonia to generate radicals 112 and 113, respectively. It should be noted that the corresponding tetrazolate anion (tetrazolide) was more resistant to irradiation (Scheme 25).
In the 1970s, Butler and co-workers[127, 128] used electron spin resonance to study the γ-irradiation-induced degradation of three series of 5-aminotetrazole derivatives: 1H-tetrazol-5-ylamines 25, N-(1-methyl-1H-tetrazol-5-yl)nitramines 110 and N-(1-methyl-1H-tetrazol-5-yl)nitrosamines 111. Methyl-substituted compounds 110 decompose to give radicals of the XCH2• и СН3• types according to Scheme 26. The degradation of nitramines 110 proceeds with the cleavage of the exocyclic N – N bond and the formation of the NO2 radical (see Scheme 26). The degradation of nitrozoamines 111 is even more complicated.
Ryabykh and Kholodkovskaya[129] showed that the radiation chemical yield (G) dependence for tetrazene 107 under γ-radiolysis conditions is described by an empirical formula:
\( G=\alpha T^{1.53} \)
where \( \alpha \) is a coefficient, \( T \) is temperature.
The authors of a study[130] also investigated the effect of ionizing radiation (radiation doses of 20 – 80 kGy,) obtained using a linear electron accelerator, on the physicochemical properties of poly(N-methylallyl-5-vinyltetrazole) and compositions based theron. It has been shown that the thermal decomposition character of the polymer exposed to high doses of radiation (up to 80 kGy) changes significantly compared to the non-irradiated sample, which may be related to the degradation of the copolymer components. The use of ionizing radiation can significantly reduce the curing time of such polymer compositions.
A large number of publication are devoted to the radiolysis of triaryltetrazolium salts 114 in various media (alcoholic, aqueous, aqueous-alcoholic solutions, solid matrices), which is of practical value. Pikaev and Kriminskaya[131] have made a significant contribution to this field of chemistry; their results are summarized in the review. The g-radiolysis of triaryltetrazolium salts 114 proceeds with high radiochemical yields to furnish coloured formazanes 115. Such radiolysis is a two-electron process, but the \( G \) value often exceeds half the number of solvated electrons, indicating that other radicals formed in the reaction medium are also involved in the transformation. For example, according to a plausible scheme confirmed by EPR, in the presence of propan-2-ol, a tetrazolium radical 116 is formed in a first step, which is further converted to formazan 115 via disproportionation (Scheme 27).
These processes can be used in the development of promising dosimetric systems, as the coloured products can be easily detected by the naked eye or quantified spectrophoto-metrically[132, 133]. The presence of oxidizing and reducing agents has a noticeable effect on this reaction[134, 135].
As mentioned above, an important characteristic of drugs is their resistance to ionizing radiation. This property determines the possibility of using the drug at high radiation levels or methods of sterilizing it using X-rays or b-radiation. Considering that the tetrazole heterocycle is highly sensitive to radiolysis, such studies seem quite relevant. For example, Sarcan et al.[136] showed that the active ingredient of the antihypertensive drug losartan (117) is resistant to X-ray radiation. The mechanism of degradation of compound 117 in aqueous solutions under g-radiolysis (doses of 0.5 – 4 kGy) was explored[137]. The degradation products of the starting compound, including the unsubstituted tetrazole 1(N1H), were determined by liquid chromatography-mass spectrometry. It was also found that the process is initially induced by the hydroxyl radical generated from water (Scheme 28).
Several papers (see e.g.,[138]) are devoted to the evaluation of the stability of the active ingredient of the semisynthetic antibiotic cefazolin (Kefzol, 118) in aqueous solutions and in the solid phase (Figure 13). The degradation of compound 118 under electron pulse radiolysis conditions (average doses of 3 – 5 Gy per 2 – 3 ns pulse) in aqueous medium is also activated by hydroxyl radical. However, the composition of the degradation products of this compound could not be determined.
The sodium salt 118 in the solid phase has proven to be very resistant to the action of g-radiation[139]. Under irradiation using a linear electron accelerator up to doses of 25 kGy, no significant degradation of this compound in the solid phase was observed either, making it safe to sterilize this drug by irradiation[140].
6. Conclusion
The processes of tetrazole decomposition initiated by thermal heating, electromagnetic or radiation irradiation and ionization under mass spectrometric conditions have now become the focus of attention of a wide range of researchers. The chemical energy stored in the tetrazole ring is released and gaseous products, mainly molecular nitrogen, are eliminated. This gives rise to highly reactive intermediates, such as the nitrile imines discovered by R.Huisgen, which can react rapidly and selectively with dipolarophiles via the 1,3-dipolar cycloaddition pathway, and also react with nucleophiles. The intensive development of this field of research is associated with the emergence of new techniques, including equipment for flash vacuum pyrolysis. Due to the pulsed temperature (up to 500 – 900 °C) and the use of vacuum technology, FVP makes it possible to control the formation of tetrazole decomposition products using spectral methods. The decomposition of tetrazoles is one of the components of the scientific concept of click chemistry and bioorthogonal reactions, the authors of which were awarded the Nobel Prize in Chemistry in 2022. This proves the relevance of the topic in relation to the formation of various tetrazole-derived products and their further transformations. At the same time, its development will largely depend on progress in the development of methods for the preparative isolation of target compounds, as well as for the performance and control of bioorthogonal reactions occurring in the cell.
The authors of this review hope that it will be of interest to a wide scientific audience.
7. List of abbreviations and designations
API — active pharmaceutical ingredient,
BTATz — 3,6-bis(1Н–1,2,3,4-tetrazol-5-ylimino)–1,2,4,5-tetrazine,
DFT — density functional theory,
DSC — differential scanning calorimetry,
es — solvated electron,
EWG — electron-withdrawing group,
FTIR — Fourier-transform infrared spectroscopy,
FVP — flash vacuum pyrolysis,
G — radiation chemical yield,
\( \Delta H_{f}^o \) — enthalpy of formation,
HAG — homoallylglycine,
mAbs — monoclonal antibodies,
MW — microwave activation,
NEM — N-ethylmaleimide,
PBS — phosphate buffered saline,
PEG — polyethylene glycol,
PET — positron emission tomography,
rt — room temperature,
SAM — self-assembled microlayers,
SMD — solvation model,
TCB — trichlorobenzene,
TGA — thermogravimetric analysis.
Acknowledgements
This review was financially supported by the Russian Science Foundation (Project No. No 23–13-00224, https://rscf.ru/project/23–13-00224/).
References
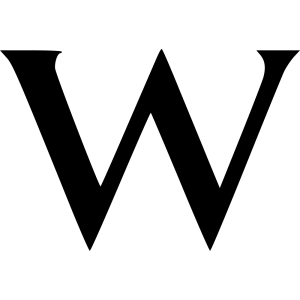
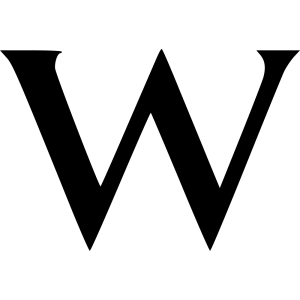
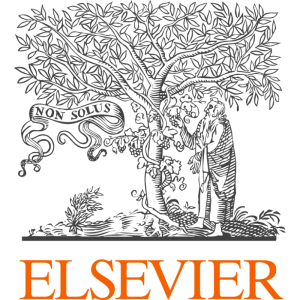
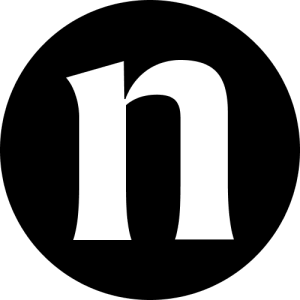
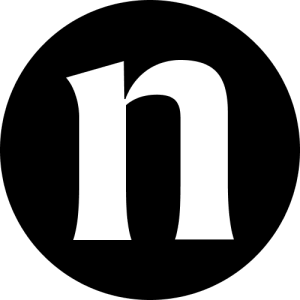
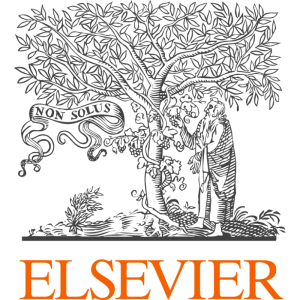
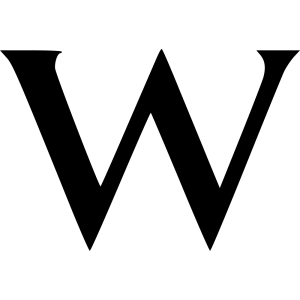
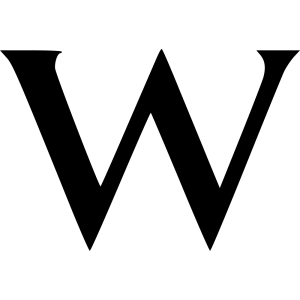
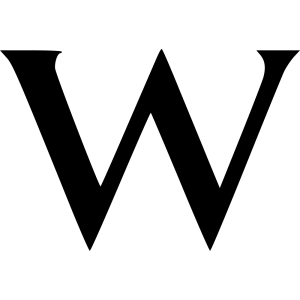
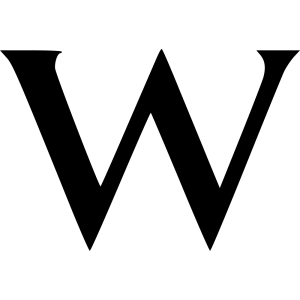
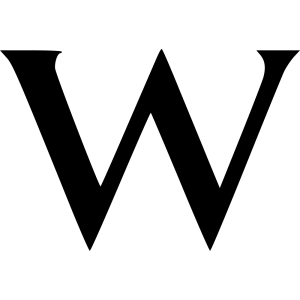
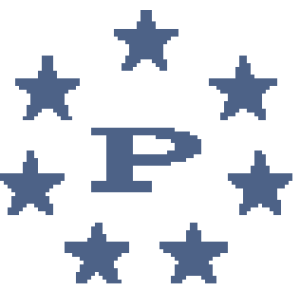
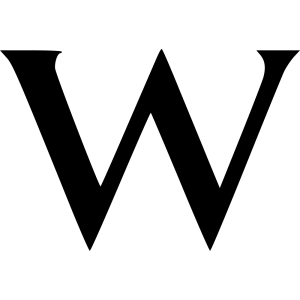
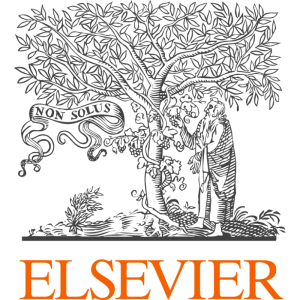
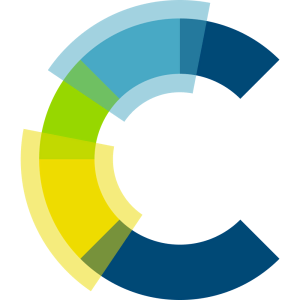
![Synthesis and properties of 5-nitro-2-[2-(3-nitro-1H-1,2,4-triazol-1-yl)ethyl]-2H-tetrazole](/storage/images/resized/voXLqlsvTwv5p3iMQ8Dhs95nqB4AXOG7Taj7G4ra_small_thumb.webp)
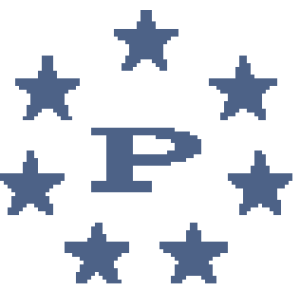
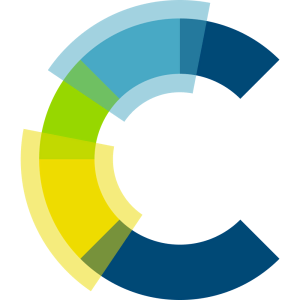
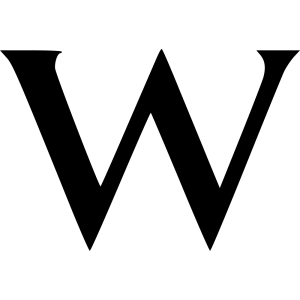
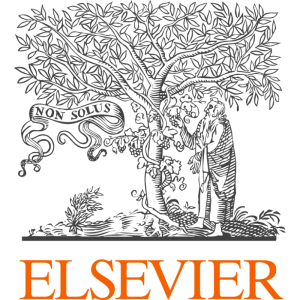
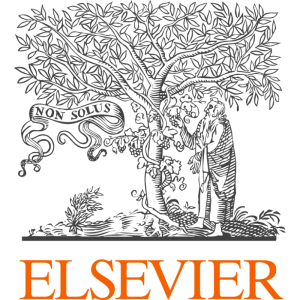
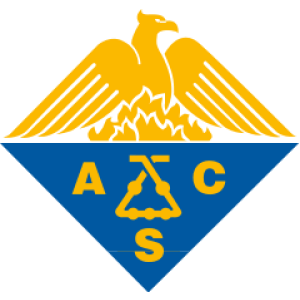
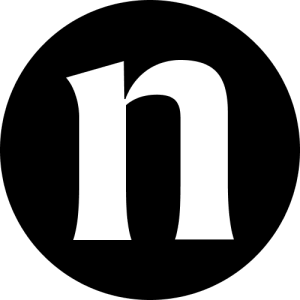
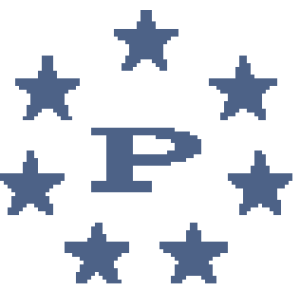
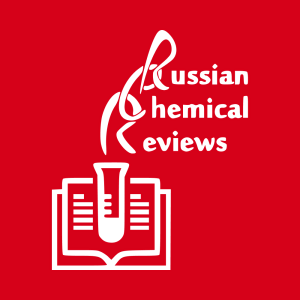
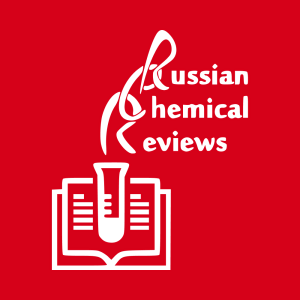
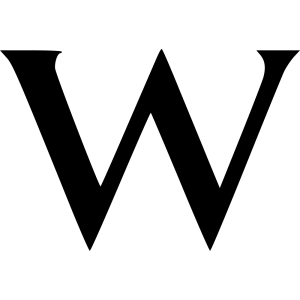
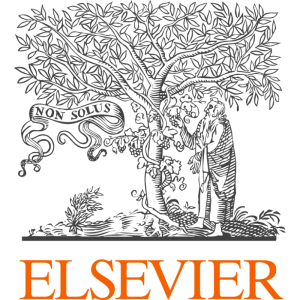
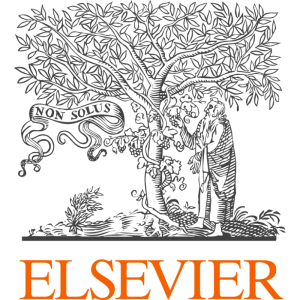
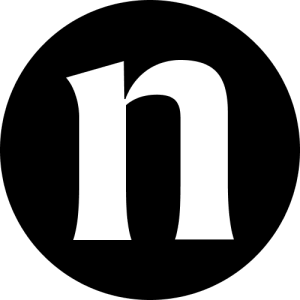
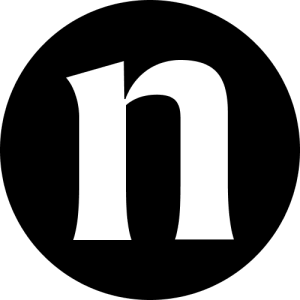
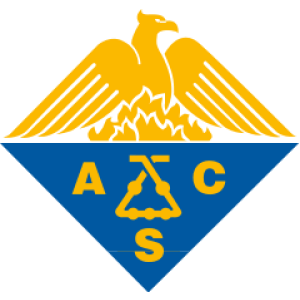
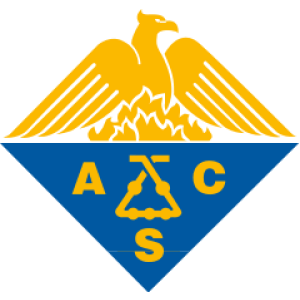
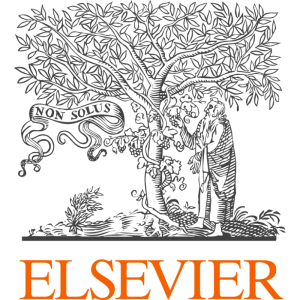
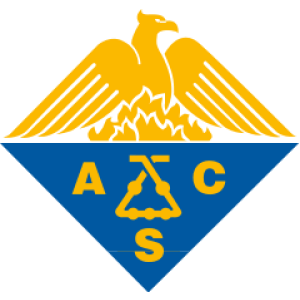
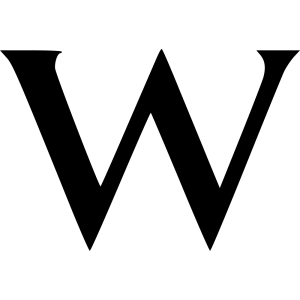
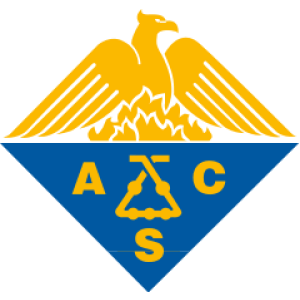
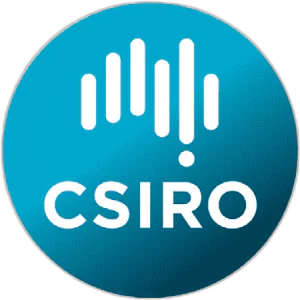
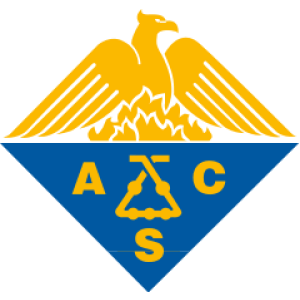
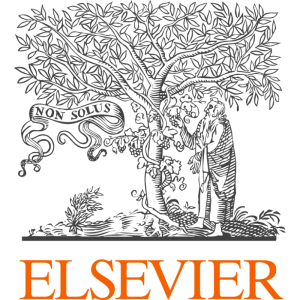
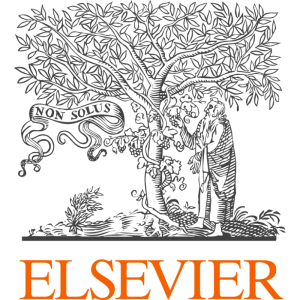
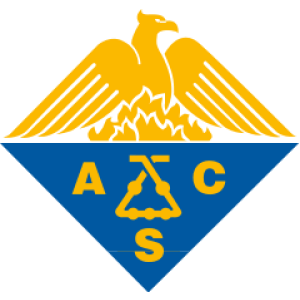
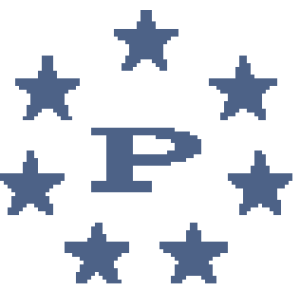
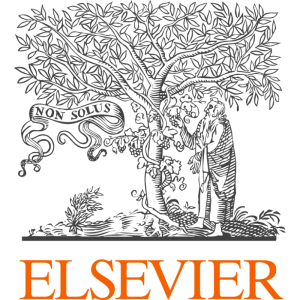
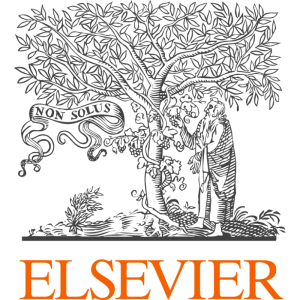
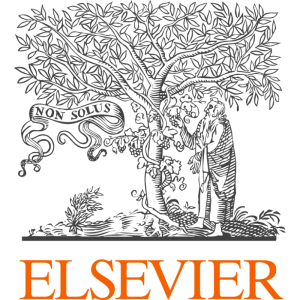
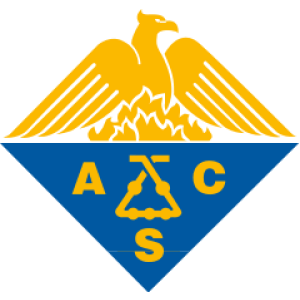
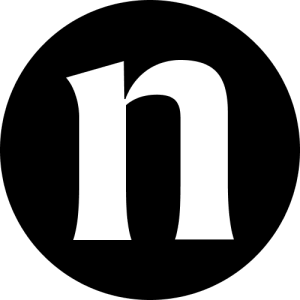
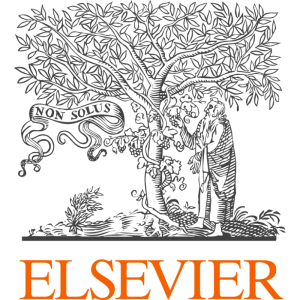
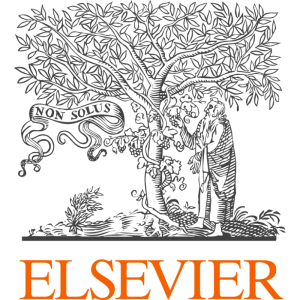
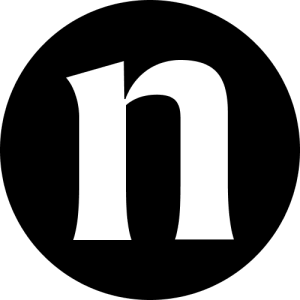
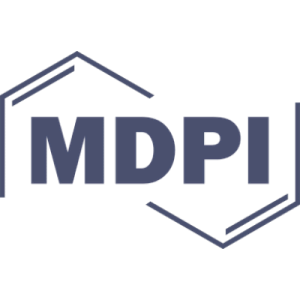
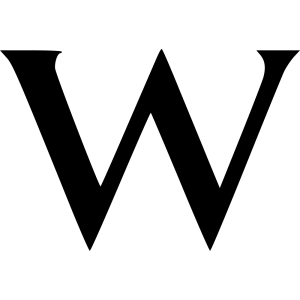
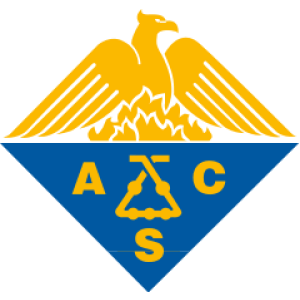
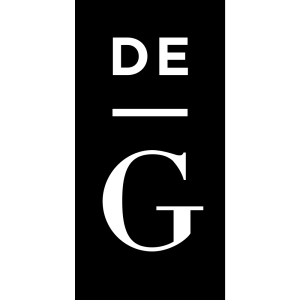
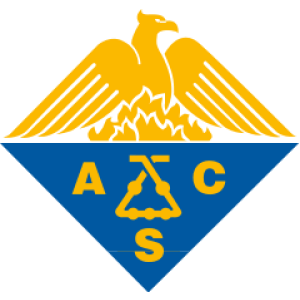
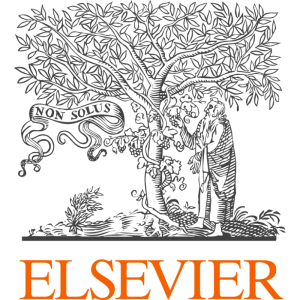
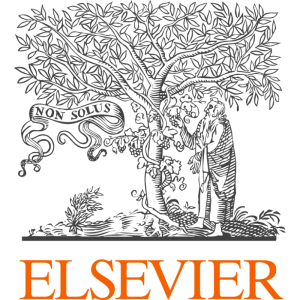
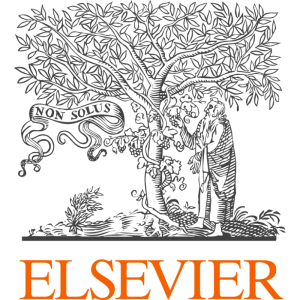
![Facile synthesis of 9H-pyrimido[4,5-b]indoles from photolysis of 8-phenyltetrazolo[1,5-c]pyrimidines in acidic media](/storage/images/resized/iLiQsFqFaSEx6chlGQ5fbAwF6VYU3WWa08hkss0g_small_thumb.webp)
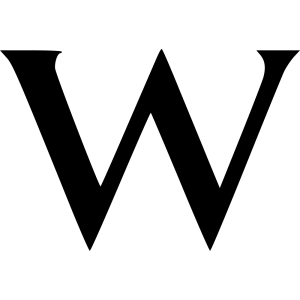
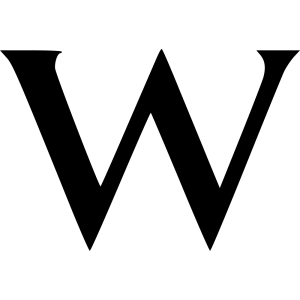
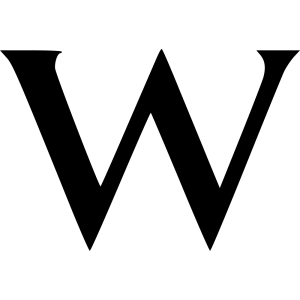
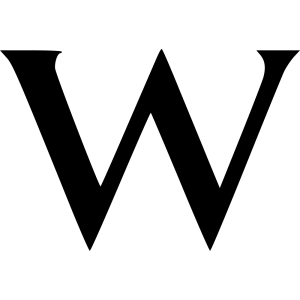
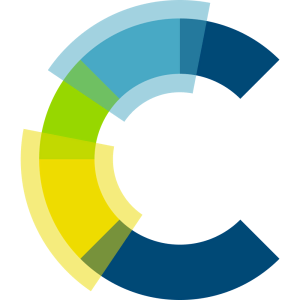
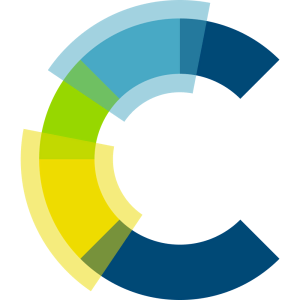
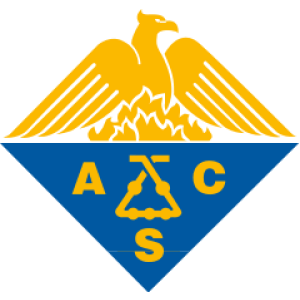
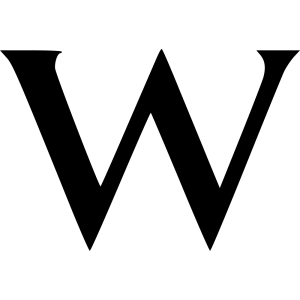
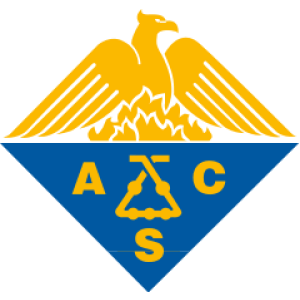
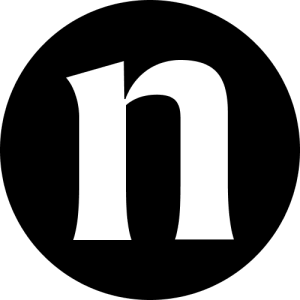
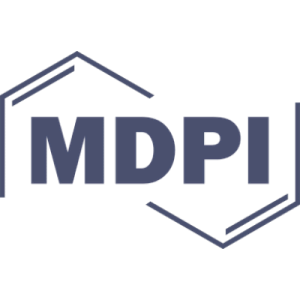
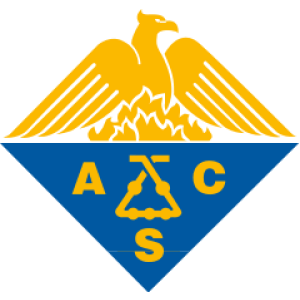
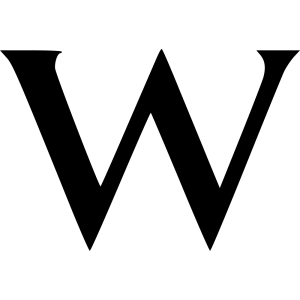
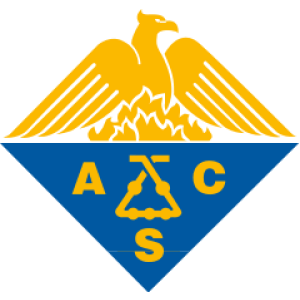
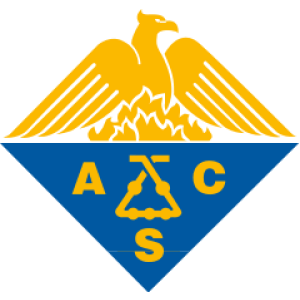
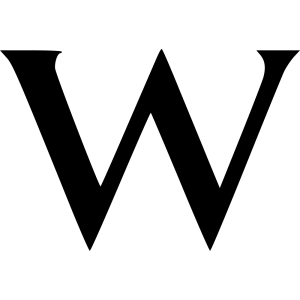
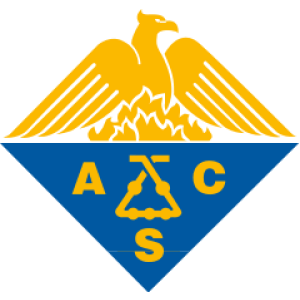
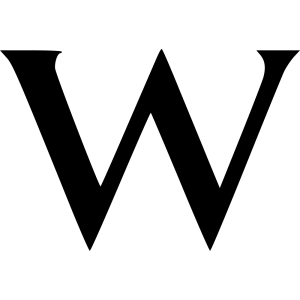
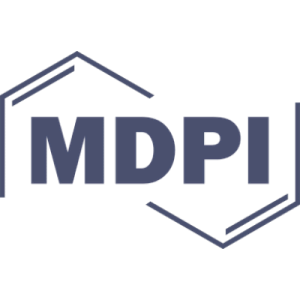
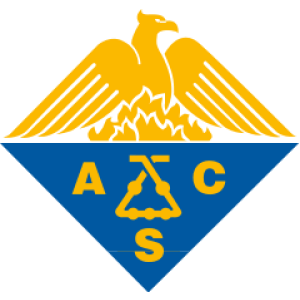
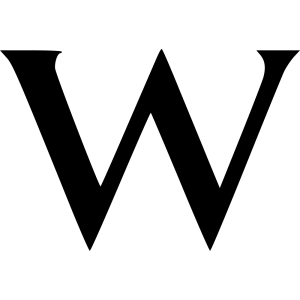
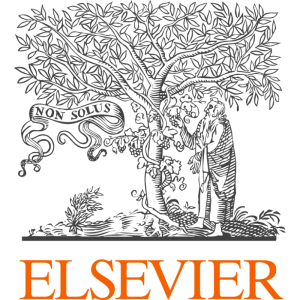
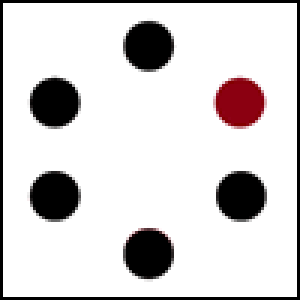
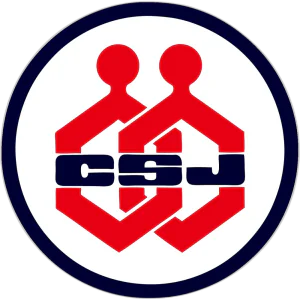
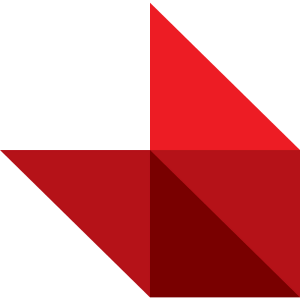
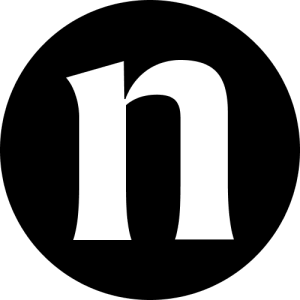
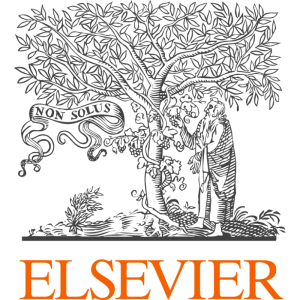
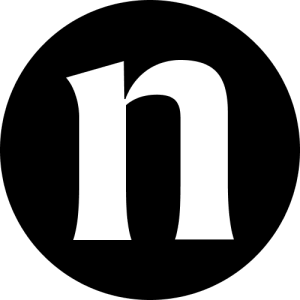
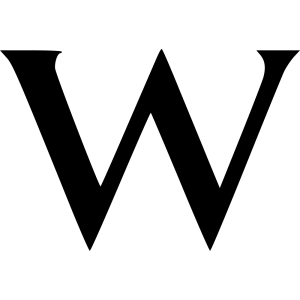
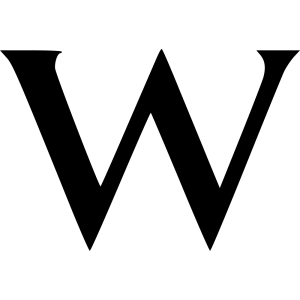
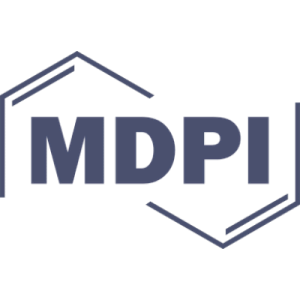
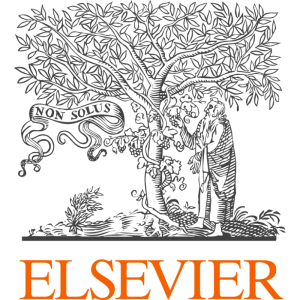
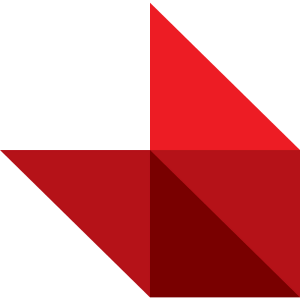
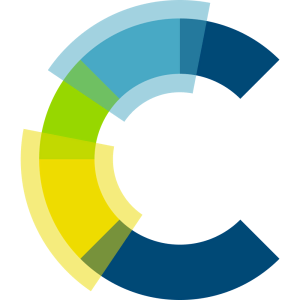
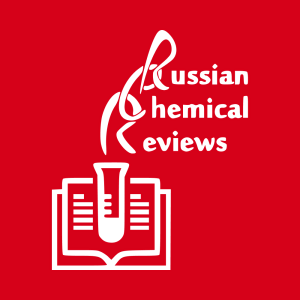
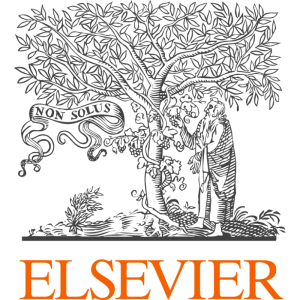
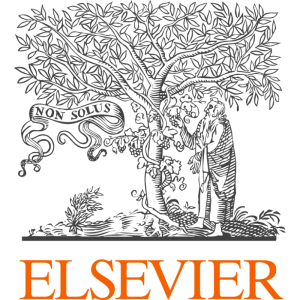
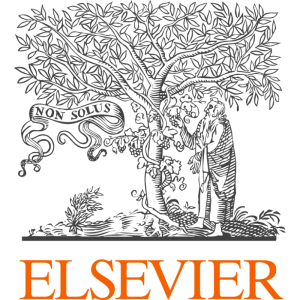
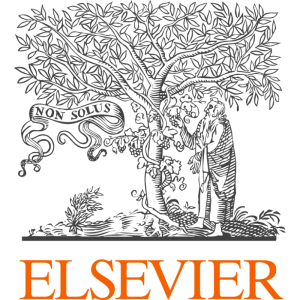
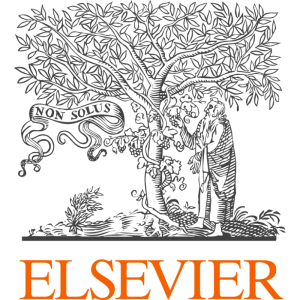
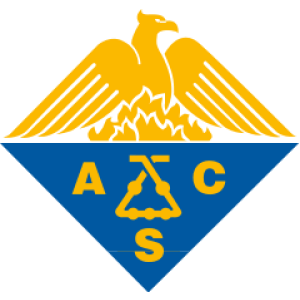
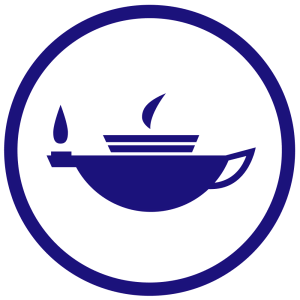
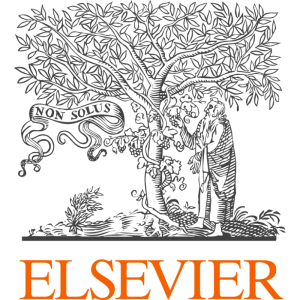