Keywords
Abstract
Chitosan, a natural and abundant polysaccharide, offers several advantageous properties, such as excellent biocompatibility, biodegradability, and antimicrobial activity, making it a highly versatile material. However, its extensive application is hindered by its insolubility in water and most organic solvents, due to numerous intramolecular/intermolecular hydrogen bonds. To overcome this limitation and optimize its benefits, chitosan is often chemically modified to enhance its utility in various industries. Biomedical materials, environmental management, biological papermaking, food preservation, and the daily chemical industry are a few examples. Modified chitosan retains the inherent properties of chitosan and acquires new physicochemical characteristics as a result of introduced functional groups. Hydrophilic modifications typically include carboxylation, phosphorylation, and quaternary ammonium modification reactions. The specific properties and applications of chitosan derivatives depend on the grafted groups. This review summarizes the reaction conditions, properties, and applications of hydrophilic chitosan derivatives in drug delivery and food preservation, serving as a reference for the development and use of chitosan-based biomaterials.
The bibliography includes 128 references.
1. Introduction
Chitosan, a deacetylation product of chitin[1], is found widely in crustacean shells (e.g., crabs and shrimp), insect exoskeletons, mollusk shells (e.g., squid), and fungal cytoderms[2-5]. Chitosan exhibits a number of biological properties, such as biocompatibility, nontoxicity, biodegradability, and antibacterial and antioxidant activities[6-8]. These advantages make chitosan an ideal material in tissue engineering[9, 10], drug delivery[11-13], food preservation[14, 15] and wound dressings[16, 17]. The physicochemical and biological properties of chitosan depend on its deacetylation degree and molecular weight. Generally, chitosan with a high degree of deacetylation and low molecular weight displays more favorable biological properties[18, 19]. However, the extensive application of chitosan is limited by the large amount of intermolecular/intramolecular hydrogen bonds, leading to poor solubility in most solvents[20].
A common strategy to overcome this disadvantage is to partially break the β-1,4 glycosidic linkages to yield low-molecular-weight chitosan or chitooligosaccharides to improve the solubility of chitosan. Chitosan degradation is typically performed using acids such as HCl, HNO3, and H3PO4. Unfortunately, the acid treatment leads to equipment corrosion and produces a large amount of acidic wastewater. Furthermore, acid hydrolysates contain a high concentration of less reactive monosaccharides and disaccharides. Therefore, chitosan is often chemically modified to overcome this disadvantage[21, 22]. During modification, hydrophilic groups, such as carboxyl, quaternary ammonium, and phosphate groups are grafted onto the chitosan skeleton[23-25]. The modified chitosan retains the intrinsic properties of native chitosan, in addition, it also possesses the unique properties of the grafted groups, such as moisture retention and film-forming ability[26].
In this review, the hydrophilic modification of chitosan, including modification methods, reaction conditions, properties, and applications of the modified chitosan, have been summarized. The prospects for the further development of this biopolymer have been discussed. This review is expected to guide the reader through the development and application of water-soluble chitosan-based biomaterials.
2. Modification of chitosan
2.1. Carboxylation modification
Carboxylation involves the grafting of carboxyl (COOH) or carboxylate groups (COO–) onto the chitosan skeleton. The water solubility of chitosan in the aqueous medium is notably increased after this modification, and a high degree of carboxyl substitution corresponds to a high solubility[27]. Carboxymethyl chitosan is one of the most common carboxylic-modified chitosan derivatives. Three types of carboxymethyl chitosan can be prepared by adjusting the reaction conditions, namely, O-carboxymethyl, N-carboxymethyl, and N,O-carboxymethyl chitosan. The amino (NH2) and hydroxyl (C(3) – OH, C(6) – OH) groups on the chitosan skeleton are the reactive sites. O-carboxymethyl and N,O-carboxymethyl chitosan are mainly prepared in a 2-C3H8O/strong alkaline medium, where –H is deprotonated into O– ion. The O– ion exhibits strong nucleophilicity[28-30]. N-carboxymethyl chitosan can be prepared by grafting glyoxylic acid in aqueous solutions, followed by reduction with sodium cyanide borohydride. The routes for the synthesis of the three types of carboxymethyl chitosan derivatives are summarized in Scheme 1.
O-Carboxymethyl chitosan with a degree of substitution DS = 0.34 was prepared in an aqueous solution of iso-C3H7OH/NaOH at 5 °C and NaOH concentration of 13.5 wt.% using monochloroacetic acid as a modifying agent[31]. The degree of substitution is the ratio of the functional groups (such as carboxymethyl in O-carboxymethyl chitosan) to the total amount of OH or NH2 groups in chitosan (here, the chitosan derivative is O-substituted, therefore, the total amount of OH refers to C(6) – OH). The percentage of available NH2 groups in the O-carboxymethyl chitosan products was found to be 78%, which was similar to that in the raw materials (79%). This finding indicates that the substitution reaction mainly involves the OH group. Temperature increase had little effect on the DS of O-carboxymethyl chitosan; for instance, when the reaction temperature was increased to 30 °C, the DS increased only slightly to 0.35[32]. In contrast, the pretreatment of chitosan significantly affected the DS of O-carboxymethyl chitosan. For example, when chitosan was pretreated by alkalization and/or freezing, while other conditions were kept constant, the DS increased significantly to 0.73[33], 0.75 (Ref. [34]) and 0.83[35]. Moreover, O-carboxymethyl chitosan with a DS of 1.2 could be prepared by alkali treatment and freezing chitosan at –20 °C for 12 h. This could be attributed to the presence of alkylated hydroxyl groups O–, which have a high electron density and strong nucleophilicity and are favorable for the SN2 substitution reactions with the positively charged C – Cl carbon atoms[36].
In addition, N-carboxymethyl chitosan can be synthesized in a strong alkaline solution via the reaction of the amino groups with the aldehyde groups, forming Schiff base, followed by reduction with sodium borohydride (NaBH4)[37]. Muzzarelli et al.[38] prepared N-carboxymethyl chitosan with a DS = 0.14 and isoelectric point of 4.1 by reacting acetic acid with amino groups, followed by reduction with sodium cyanoborohydride (NaBH3CN) at room temperature and alkaline pH. Miranda et al.[39] prepared N-carboxymethyl chitosan with a DS = 0.185 using sodium borohydride, and the resulting N-carboxymethyl chitosan showed higher thermal stability than chitosan. The DS of N-carboxylated chitosan can be tuned by varying the amount of reactants, temperature, and the pH of the reacting medium[40].
With unprotected NH2 and C(6) – OH functionalities, the grafting reactions can occur simultaneously on both groups under certain reaction conditions, resulting in the formation of N,O-substituted carboxymethyl chitosan. NaOH or KOH/2-propanol solutions are commonly used media for the preparation of N,O-carboxymethyl chitosan. For instance, N,O-carboxymethyl chitosan with a total DS of 0.245 was prepared in a NaOH/isopropanol mixture with a mass ratio of chitosan to monochloroacetic acid of 1/6 and a temperature of 60 °C, and the DS values of NH2 and OH groups were 0.135 and 0.10[41], respectively. This result indicates that the reactivity of NH2 is slightly higher than that of C(6) – OH in NaOH/2-propanol solution. This finding was confirmed by the synthesis of N,O-carboxymethyl chitosan with total DS values of 0.3 (mchitosan/mmonochloroacetic acis = 1/6) and 0.5 (mchitosan/mmonochloroacetic acis = 1/30) at a reaction temperature of 65 °C for 3 h, respectively. The DS values of NH2 were 0.175 and 0.3 and those of the C(6) – OH groups were 0.125 and 0.2[42], respectively.
Reaction temperature had a significant effect on DS, and a relatively low reaction temperature favoured the formation of chitosan derivatives. For example, the DS of N,O-carboxymethyl chitosan prepared at 60 °C was 1.59[43], which was higher than that of chitosan prepared at 65 °C[42]. Intermittent operation could also significantly improve the DS of N,O-carboxymethyl chitosan. Cheng et al.[44] synthesized N,O-carboxymethyl chitosan with a DS of 1.68 in NaOH/2-propanol solution by the intermittent addition of chloroacetic acid (at a frequency of every 5 min). The researchers also showed that the reaction occurred mainly at C6-OH to give a DS = 0.86, followed by the reaction at NH2 to give a DS = 0.82. The high electronegativity and low steric hindrance of C(6) – O– are thought to play a key role in the formation of O-carboxymethyl chitosan.
2.2. Phosphorylation modification
Phosphorylated chitosan is another commonly used anionic derivative of chitosan. Moreover, phosphate ions can impart desirable biological activities to the chitosan derivative, including antitoxic, antibacterial, antioxidant, and enzyme inhibitory activities[45-47]. H3PO4 (Ref. [48]) and P2O5 (Ref. [49]) are the most commonly used in this reaction.
When H3PO4 is used in the phosphorylation reaction, it reacts with NH2 groups; however, in a weak acid medium, P2O5 reacts with OH groups[50]. The water solubility of phosphorylated chitosan derivatives depends on their DS. A high DS corresponds to an increased water solubility of the phosphorylated chitosan. The DS depends on several factors, such as reaction temperature and time and the H3PO4 (or P2O5): NH2 mass ratio. Phosphorylated chitosan with a DS = 0.37 was prepared in a dilute acetic acid solution by grafting H3PO4 onto the polymer at 5 °C[51]. In contrast, phosphorylated chitosan with DS of 0.6 – 0.98 was prepared at 5 °C by adjusting the reaction time and the amount of P2O5 in the chitosan/P2O5/mesylate medium[52]. When H3PO4 and P2O5 were used simultaneously to modify chitosan, the DS of the modified chitosan was almost equal to that of chitosan modified by H3PO4 or P2O5 alone, due to the mutual repulsion between H3PO4 and P2O5. Amaral et al.[53] and Ma et al.[54] confirmed these results by modifying chitosan in a H3PO4/Et3PO4/P2O5/2-butanol mixture (DS = 0.2) and an ethanol/triethylamine/CCl4/H2O mixture by the Atherton-Todd reaction in a one-step process (DS = 0.1) (Scheme 2).
2.3. Quaternary ammonium modification
Quaternary ammonium chitosan is a type of cationic chitosan derivative prepared in two ways (Scheme 3). One of them (see Scheme 3, reaction 1) involves the direct introduction of quaternary ammonium groups onto the chitosan skeleton (C(6) – OH or NH2) and the other (see Scheme 3, reactions 2 – 4) is the multistep quaternization of NH2 groups[55]. Quaternary ammonium chitosan derivatives have additional properties, such as improved antibacterial activity and good water solubility, which are independent of factors such as pH of a solution.
Theoretically, C(6) – OH, C(3) – OH, and NH2 groups are all reactive sites, but C(3)–OH exhibits the greatest steric hindrance, and hence, the least reactivity. In a neutral medium, the reactivity of the C(6) – OH and NH2 groups is almost equal. Therefore, when using the direct introduction method, it is necessary to protect one of the active groups to obtain single-group substituted quaternary ammonium chitosan (either C(6) – OH or NH2). Once the quaternary ammonium moiety is introduced, the protected groups are removed. For example, O-quaternary ammonium chitosan derivatives with DS of 0.4 and 0.3 were prepared by protecting the NH2 groups with benzaldehyde[56] and 4-nitrobenzaldehyde[57], respectively, with subsequent removal of protecting groups. This method is commonly used to prepare O-substituted quaternary ammonium chitosan, which forms the Schiff base with NH2 and is then deprotected. O-substituted quaternary chitosan could also be prepared by grafting the quaternary group onto the C(6) – OH group by increasing its selectivity. Du et al.[58] grafted glycidyl trimethylammonium chloride with a DS = 0.39 onto C(6) – OH in an 2-propanol/NaOH medium. In a strong base solution, the C(6) – OH group is neutralized to C(6) – O−, which is much more reactive than the C(3) – OH and NH2 groups. All quaternary ammonium groups containing epoxides, halogen atoms, acyl groups and anhydrides can be grafted onto the C(6) – OH of chitosan skeleton using this procedure[59]. In addition, quaternary ammonium chitosan can be prepared by the reaction of active functional groups with other substances to form a highly reactive intermediate that reacts with the group being introduced. For instance, Wang et al.[60] introduced 1-(4-carboxyphenyl)-N,N,N-trimethylammonium onto chitosan with activation by 1-ethyl-3-(3-dimethylaminopropyl) carbodiimide and N-hydroxysuccinimide to produce N-quaternary ammonium chitosan with DS = 0.2.
Quaternary ammonium chitosan can also be prepared by multiple oxidation of NH2 groups. In the substitution reaction, the reactivity of NH2 is higher than that of C(6) – OH and C(3) – OH; therefore, the products of NH2-substitution can be prepared under appropriate reaction conditions. For instance, Rúnarsson et al.[61] obtained N,N,N-trimethylammonium chitosan with DS ≥ 0.7 using the multiple iodomethane substitution method. N-quaternary ammonium chitosan displayed stronger antibacterial activity than chitosan. Pardeshi et al.[62] prepared N,N,N-trimethyl iodide chitosan with DS = 0.67 in sodium iodide/N-methyl-2-pyrrolidone using the multiple reaction method.
In recent decades, the development of ionic liquids has attracted considerable attention. Ionic liquids have been reported to successfully dissolve polysaccharides such as chitosan, chitin, and cellulose[63-65], and can therefore be used as green solvents in the homogeneous modification of these polysaccharides[65, 66]. Generally, NH2 is more reactive than OH in ionic liquids. Wei et al.[67] prepared N-1-carboxybutyl-3-methylimidazolium acyl chitosan in 1-butyl-3-methylimidazole chloride (BmimCl). In our previous work, N-[(2-hydroxyl)-propyl-3-trimethylammonium]chitosan chloride with a DS as high as 0.47 was prepared in 1-allyl-3-methylimidazolium chloride (AmimCl). Optimum reaction conditions included a duration of 8 h, a temperature of 80 °C, and a molar ratio of 2,3-epoxypropyltrimethylammonium chloride (EPTAC) to NH2 (nEPTAC/nNH2) of 3/1. Pretreatment of chitosan with dilute HCl solution increased the DS to 1.18. 2,3-Epoxypropyl trimethylammonium was grafted onto NH2 via the SN1 substitution reaction[68], and the activation energy of the reaction was 42.6 kcal mol−1 (see [69, 70]). The nucleophilic substitution reaction followed the first-order reaction rule[68], and the kinetic equation was
The relationship between the reaction constant and temperature was
Inspired by this reaction, Li et al.[71] introduced the 2,3-epoxypropyl trimethylammonium group onto the NH2 moiety of O-carboxymethyl chitosan in AmimCl to obtain an amphoteric chitosan derivative (Scheme 4), which exhibited good antibacterial activity, gel-forming ability, and low-temperature resistance.
In certain ionic liquids, the difference in reactivities of the NH2 and OH groups of chitosan is leveled down, enabling the preparation of N,O-chitosan derivatives. For example, N,O-polycaprolactone-g-chitosan was prepared in 1-ethyl-3-methylimidazolium acetate (EmimAc)[72], N,O-carboxymethyl chitosan with DS = 0.63 was prepared in 1-dodecyl-3-methylimidazolium bromide (C12MIMBr)[73, 74], and 2,2'-azino-bis(3-ethylbenzothiazoline)-6-sulfonic acid was simultaneously grafted onto the NH2 and OH groups of chitosan in 1-(3-aminopropyl)-imidazole[66, 75].
3. Application of hydrophilic modified chitosan
Compared with native chitosan, modified chitosan derivatives possess unique physicochemical properties, such as improved film-forming and gel-forming abilities, antioxidant activity, antibacterial activity, antifungal activity, and biodegradability. Therefore, these derivatives have potential applications in the biomedical field[76-79].
3.1. Biomedical applications
Hydrophilic-modified chitosan is among the most suitable candidates for gel dressings owing to its good gel-forming properties. For instance, N,O-carboxymethyl chitosan combined with oxidized chondroitin sulfate can form hydrogels with stable performance, excellent bacteriostatic ability, and most importantly, excellent hemostatic properties that are conducive to hemostatic dressing[80, 81]. Carboxymethyl chitosan/doxorubicin (DOX)-loaded gelatin nanoparticle hydrogels (DOX-NPs-Gel) exhibit pH-responsive properties in both drug release and self-degradation behavior, which could improve the penetration of DOX hydrogels into tumours and prolong their retention compared to free DOX (DOX-gel). The release rates of DOX from both DOX-Gel and DOX-NPs-Gel were determined by placing dialysis bags (14 kDa) containing DOX-loaded hydrogels in phosphate buffer solutions at pH of 7.4, 6.5 and 5.0, respectively, at 37 °C. The release rates of free DOX were 87.0% (pH = 5.0), 69.0% (pH = 6.5) and 36.5% (pH = 7.4), respectively. However, the release rates of DOX from DOX-NPs-Gel decreased significantly to 65.0% (pH = 5.0), 49.3% (pH = 6.5) and 29.9% (pH = 7.4) in 144 h, which meant that the DOX-NPs-Gel could provide a longer drug supply period. The DOX-release rates from DOX-Gel and DOX-NPs-Gel at different intervals are summarized in Table 1[80]. The drug encapsulation and release of hydrogels are shown in Figure 1. This pH-responsiveness is advantageous for targeted drug release and improved drug efficacy. In a weakly acidic environment, the hydrogels degrade slowly, thus effectively promoting the accumulation of DOX at the tumour site. N,O-carboxymethyl chitosan hydrogels prepared by the cross-linking of oxidized alginate and tricalcium β-phosphate exhibit rapid gel formation, high porosity, connectivity of the porous structure, good degradability, high compressive strength, and high in vitro cytocompatibility, which may promote wound healing[70].
Other hydrophilic chitosan derivatives, such as phosphorylated and quaternary ammonium chitosan, are also widely used in biomedical applications. Quaternary ammonium chitosan/polyvinyl alcohol-polyethylene oxide hydrogels have a uniform connected pore structure, thereby meeting the requirements for wound dressings. N,N,N-trimethyl chitosan can significantly promote wound epithelialization and contraction; therefore, hydrogels containing this derivative can be used as wound dressings and targeted drug carriers[82]. Phosphorylated chitosan is widely used in bone defect therapy due to its significant biological activity. Li et al.[83] prepared a polyelectrolyte complex using phosphorylated chitosan as a polyanion and chitosan as a polycation, which could encapsulate low-crystalline calcium phosphate owing to its regular porous structure and strong ability to bind to Ca2+ (see [83]). The polyelectrolyte complex could serve as a potential bone replacement material with controlled drug release. Glycol-modified chitosan could encapsulate as high as 94.9% eumelanin (drug loading efficiency is 204.7%), which was released in 264 hours[75]. More information about the drug loading of hydrophilic-modified chitosan is shown in Table 2, including chitosan derivative species, drug name, drug encapsulation efficiency (DEE), drug loading efficiency (DLE), pH response value of drug release (pH), and drug release rate (DRR). The results suggest that carboxymethyl chitosan is a promising drug carrier.
3.2. Food preservation
Based on the technology of drug encapsulation, the hydrophilic chitosan can be used in food preservation. Carboxymethyl chitosan-based films have been shown to be effective in extending the shelf life of enoki mushrooms[96], blueberries, and strawberries (7 days)[97], fresh apple slices (12 days)[98] and frozen meat (10 days)[99]. This effect could be attributed to the good film-forming ability, antibacterial and antioxidant properties, water vapour and oxygen blocking capability, tensile strength, flexibility, and heat resistance of the complex films. Gelatin-grafted chitosan/pomegranate peel extract films could extend the shelf life of strawberries to 8 days[100]. The permeability of chitosan-based films to oxygen has been reported to be ≤ 1% of that of carbon dioxide[101].
Quaternary ammonium chitosan derivatives are also used in food preservation because of their strong antibacterial property, water solubility, and film-forming ability. 5-Chitosan modified with 5-carboxypentyltriphenylphosphonium group (quaternary ammonium compound) has been shown to protect mango and papaya from aging for 12 days[102]. Catechol-functionalized quaternary ammonium chitosan films could effectively maintain the appearance, colour, and hardness, and extend the shelf life of strawberries and bananas to 7 and 10 days, respectively, keeping their weight and soluble substances almost constant[103]. The variation of parameters including weight loss, hardness, total soluble solid, yellowness, chroma analysis value, and titratable acidity of the strawberries coated with 2,3-epoxypropyl trimethyl ammonium chloride grafted chitosan (Q-CS) and 3,4-dihydroxy benzaldehyde grafted Q-CS (CQ-CS) in 7-day shelf life are shown in Table 3. Quaternary ammonium chitosan/tannic acid films could extend the shelf life of passion fruit to 10 days by shielding from light, oxidation, water evaporation and bacterial invasion. The coated passion fruit remained intact on the 20th day, and the weight loss rate on the 21st day was very small (Table 4)[104]. The preserving mechanism of chitosan-based film on fruits is shown in Figure 2.
In our previous studies, N-[(2-hydroxyl)-propyl-3-trimethyl-ammonium] and N-2-hydroxylpropyl-3-trimethylammonium-O-carboxymethyl chitosan were successfully synthesized[69, 105]. N-[(2-hydroxyl)-propyl-3-trimethylammonium] chitosan/carboxymethyl cellulose and N-[(2-hydroxyl)-propyl-3-trimethyl-ammonium]-O-carboxymethyl chitosan/carboxymethyl cellulose films demonstrated excellent properties, including tight structure, strong tensile strength, and low vapour and oxygen permeability[106, 107]. The N-[(2-hydroxyl)-propyl-3-trimethylammonium]-O-carboxymethyl chitosan/carboxymethyl cellulose film could effectively extend the shelf life of fresh pork to 72 h at 40 °C[108]. This effect could be attributed to the intermolecular electrostatic interaction and hydrogen bonding between the two types of polyelectrolytes, as well as the intramolecular interaction in amphoteric N-[(2-hydroxyl)-propyl-3-trimethylammonium]-O-carboxymethyl chitosan.
N-2-Hydroxypropyl-3-butyl ether-O-carboxymethyl chitosan (HBCC) and N-2-hydroxypropyl-3-(2-ethylhexyl glycidyl ether)-O-carboxymethyl chitosan (H2ECC) films crosslinked with genipin and glutaraldehyde demonstrate several desirable properties, such as low water vapour transmittance, strong water resistance, strong UV resistance, good hydrophilicity, strong antibacterial activity, and tensile strength[109, 110]. The crosslinked HBCC and H2ECC films could extend the fresh life of bananas and strawberries to 10 d (Figure 3a) and 5 d (Figure 3b), respectively. The weight losses of the bananas and strawberries were only 12% and ca. 20%, respectively, which were much lower than those of the untreated fruits. Total water-soluble sugar and malondialdehyde content in bananas[110, 111] and the level of vitamin C (Vc) in strawberries[109, 110] increased with increasing shelf life, but the rate of increase was much lower than in controls. Several reports have been published on hydrophilic modified chitosan-based materials used in the preservation of fruits, vegetables and meat, as shown in Table 5. From these it can be concluded that the genipin is less toxic than glutaraldehyde, although at high concentrations it can cause irritation and sensitization in some individuals.
4. Conclusion and prospects
In this review, we summarized the methods for modifying chitosan to make it more hydrophilic, the properties of the modified chitosan and its applications in drug packaging and delivery and fruit, food, and meat preservation. The reaction conditions, including the amount of reactants, reaction time and temperature, and catalyst type and amount affect the reaction site as well as the DS of chitosan. Hydrophilic-modified chitosan inherits the advantages of the native polymer and the grafted groups give chitosan new properties, widening its range of applications.
However, several challenges are yet to be overcome to realize the full application potential of hydrophilic-modified chitosan in biomedical and food preservation fields. (1) The safety of the reactants: the reactants and hydrophilic-modified chitosan must be guaranteed nontoxic to humans. It is therefore necessary to conduct additional comprehensive toxicity studies using the modified chitosan. (2) When hydrophilic-modified chitosan is used for drug delivery, it is difficult to achieve high encapsulation efficiency and precise conditional control of target release. (3) When used as a fruit and food preservation material, the hydrophilic-modified chitosan lacks the ability as an indicator to ensure food quality in real-time. Although studies have reported the pH-responsive property of chitosan as an indicator of food quality, the discolouration pH value of this indicator is too wide and the colour change is not obvious enough to provide accurate information. (4) Studies on the effect of chitosan on human organs are lacking, and the pathological and nutritional aspects of this biopolymer should be further investigated. (5) Compared to petroleum-based materials, the manufacturing cost of hydrophilic modified chitosan is high, which is a key factor limiting its popularization.
With the concepts of ‘carbon neutrality’ and ‘carbon peak’ gaining attention globally, the application of chitosan, the second most abundant natural polysaccharide, in the fields of drug delivery and food preservation warrants greater attention.
5. List of abbreviations
AmimCl — 1-allyl-3-methylimidazolium chloride,
BmimCl — 1-butyl-3-methylimidazolium chloride,
CQ-CS — catechol-modified quaternized chitosan,
DEE — drug encapsulation efficiency,
DLE — drug loading efficiency,
DOX — doxorubicin,
DRR — drug release rate,
DS — degree of substitution,
HBCC — N-2-hydroxypropyl-3-butyl ether-O-carboxy-methyl chitosan,
H2ECC — N-2-hydroxypropyl-3-(2-ethylhexyl glycidyl ether)-O-carboxymethyl chitosan,
PE — preservation effect,
Q-CS — quaternized chitosan
Vc — vitamin С.
Acknowledgements
The authors acknowledge the financial support from the Key Innovation Project of Qilu University of Technology and Shandong Academy of Sciences (2022JBZ02-04), Shandong Provincial University Students Innovation and Entrepreneurship Training Program (S202310431098), and the support from Russian Science Foundation (grant 20-13-00247) (Rawil Fakhrullin).
References
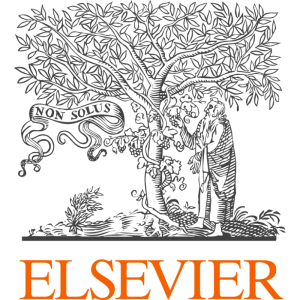
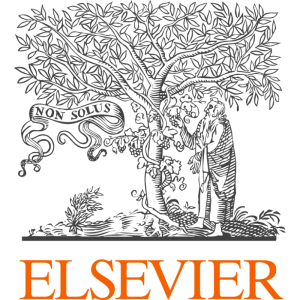
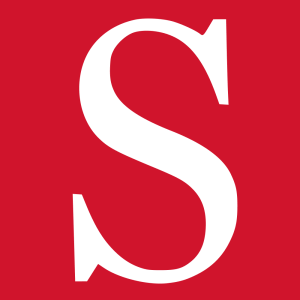
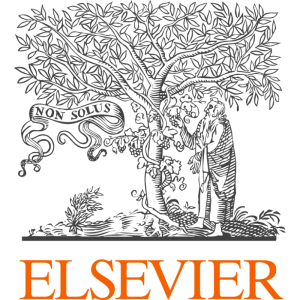
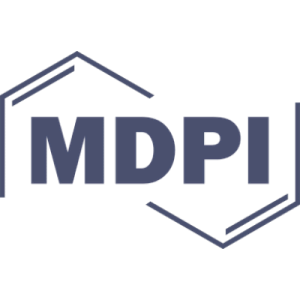
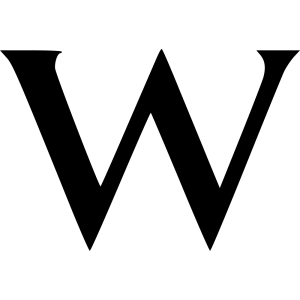
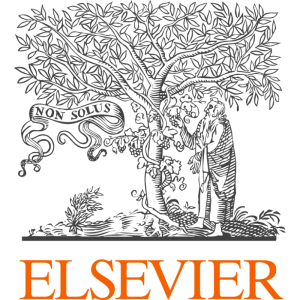
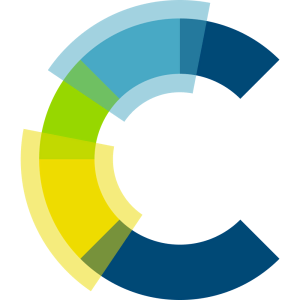
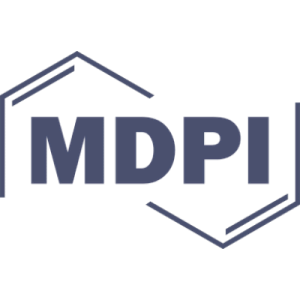
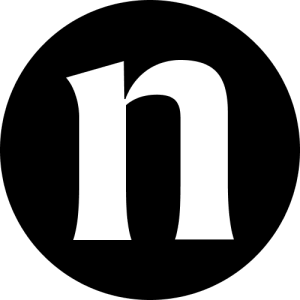
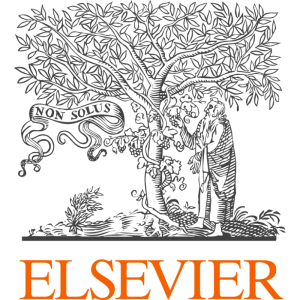
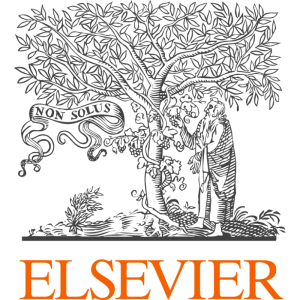
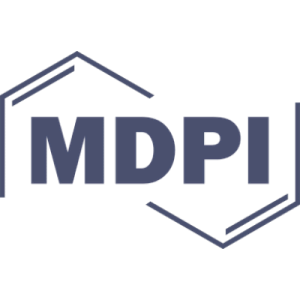
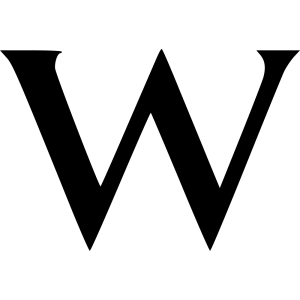
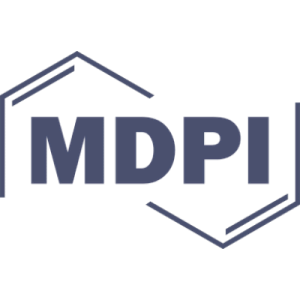
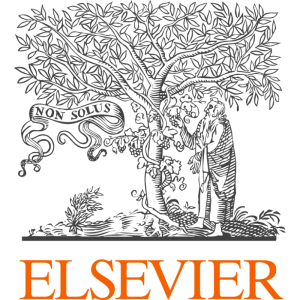
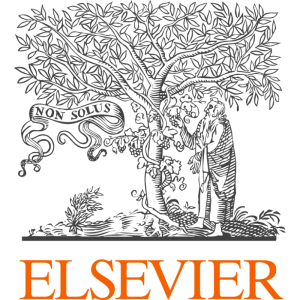
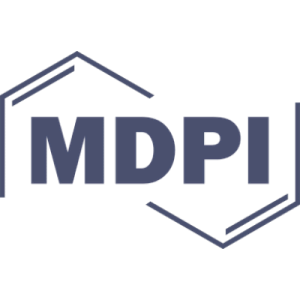
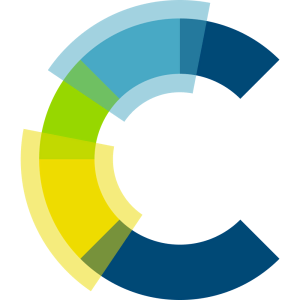
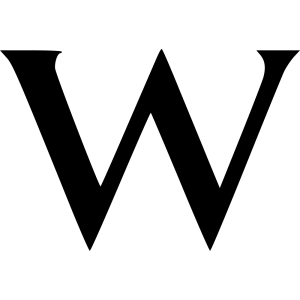
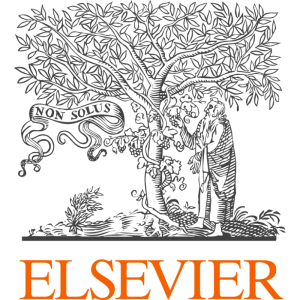
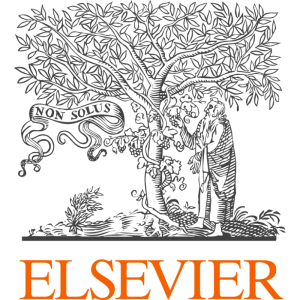
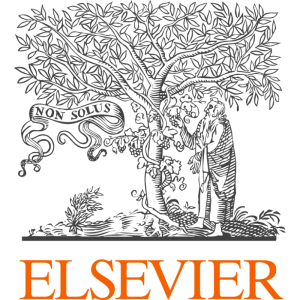
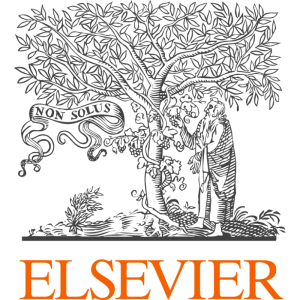
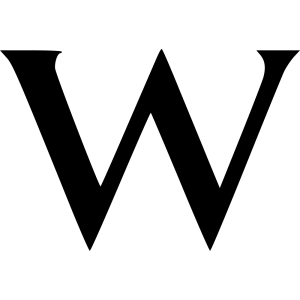
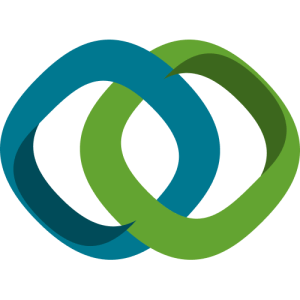
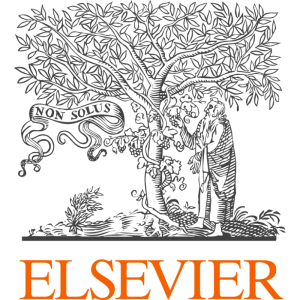
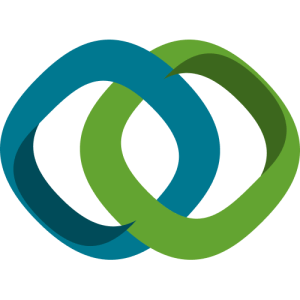
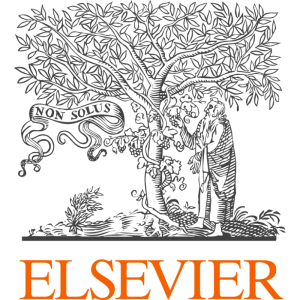
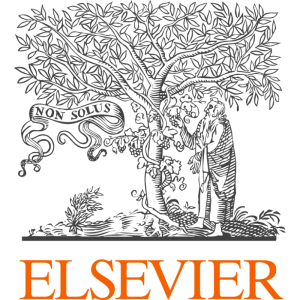
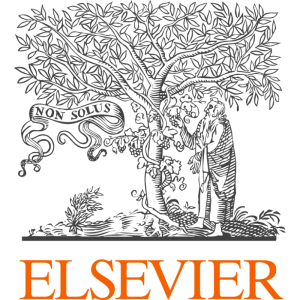
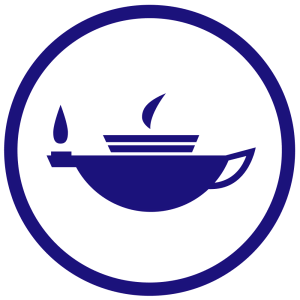
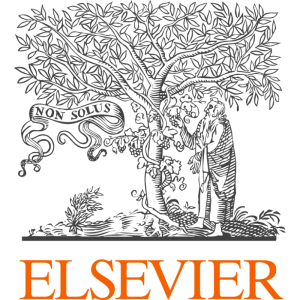
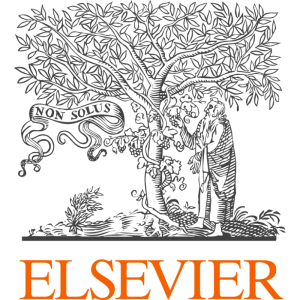
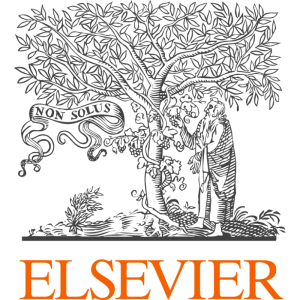
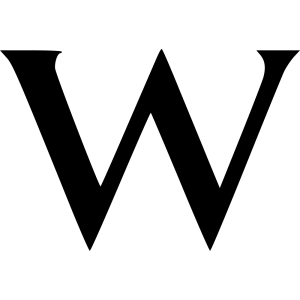
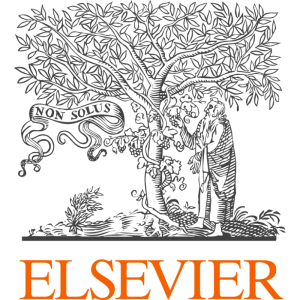
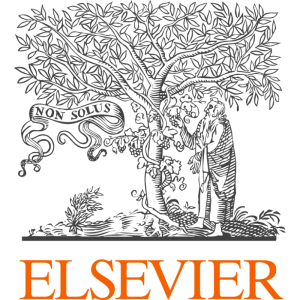
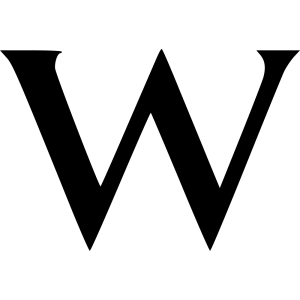
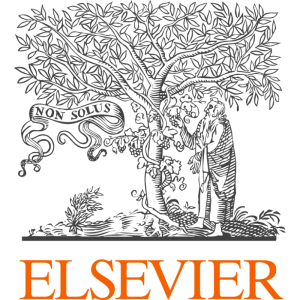
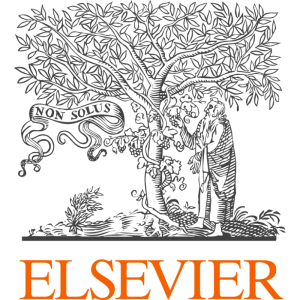
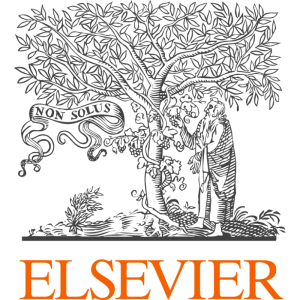
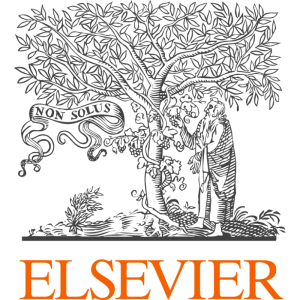
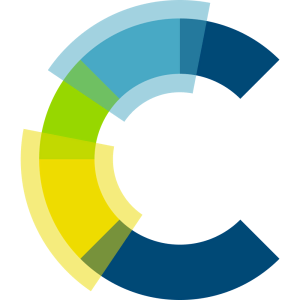
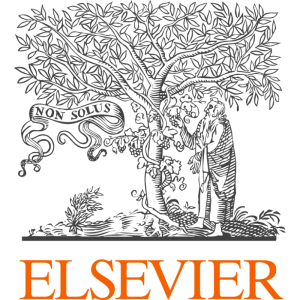
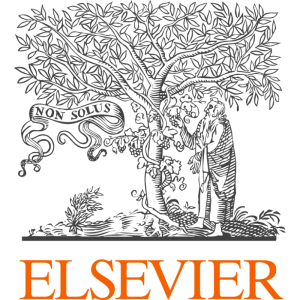
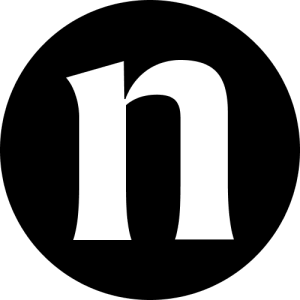
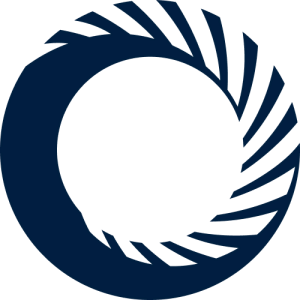
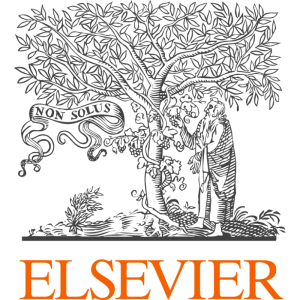
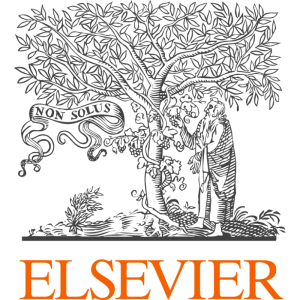
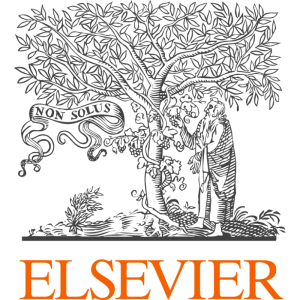
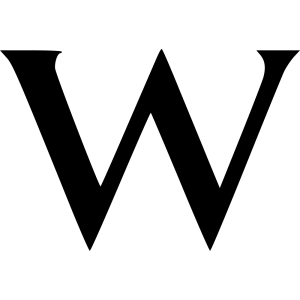
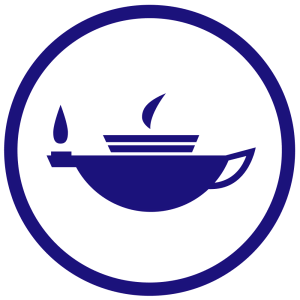
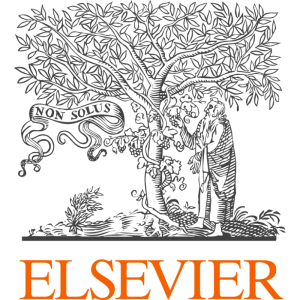
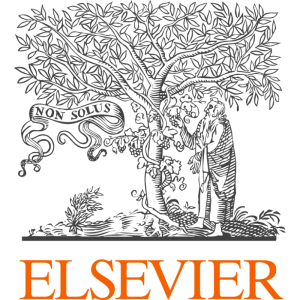
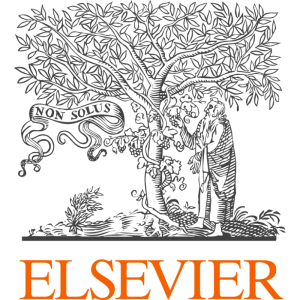
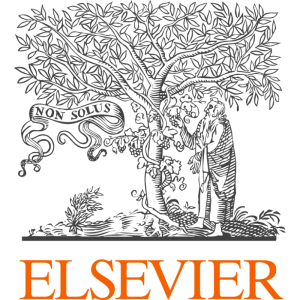
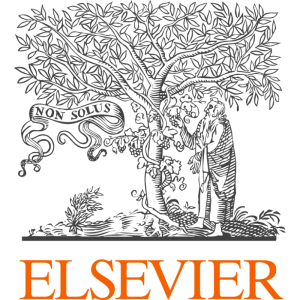
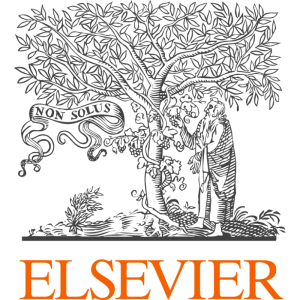
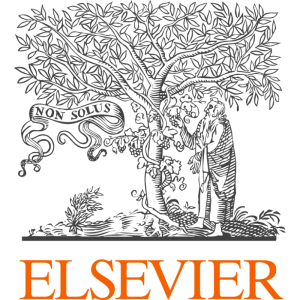
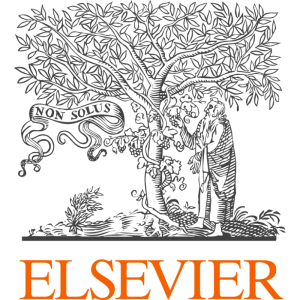
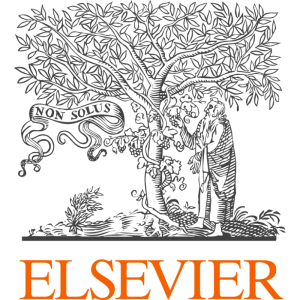
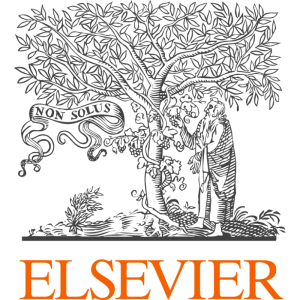
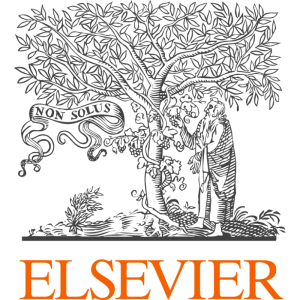
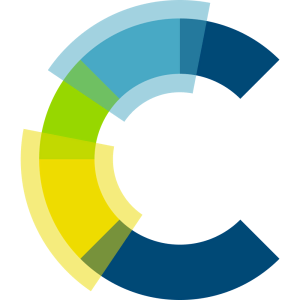
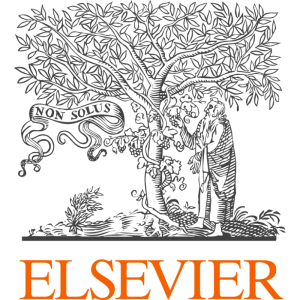
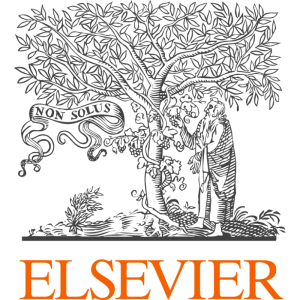
![Rheological properties of N-[(2-hydroxyl)-propyl-3-trimethyl ammonium] chitosan chloride](/storage/images/resized/GDnYOu1UpMMfMMRV6Aqle4H0YLLsraeD9IP9qScG_small_thumb.webp)
![A simple and convenient method to synthesize N-[(2-hydroxyl)-propyl-3-trimethylammonium] chitosan chloride in an ionic liquid](/storage/images/resized/GDnYOu1UpMMfMMRV6Aqle4H0YLLsraeD9IP9qScG_small_thumb.webp)
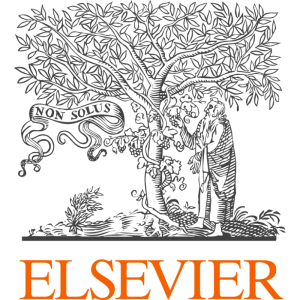
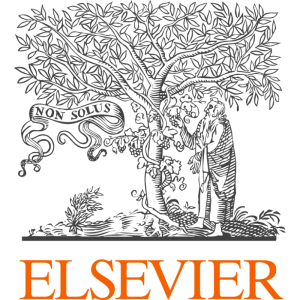
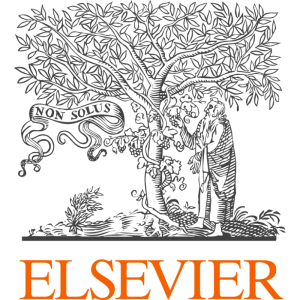
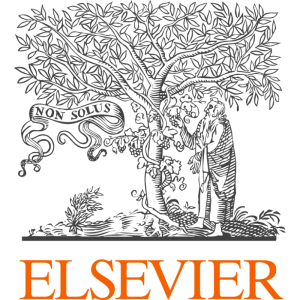
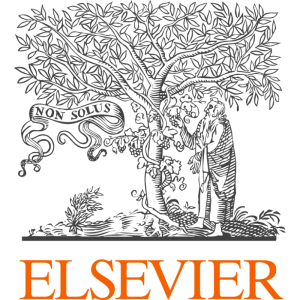
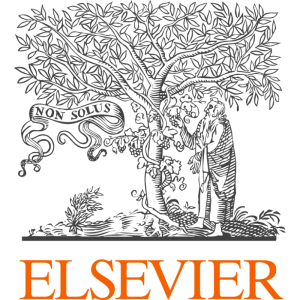
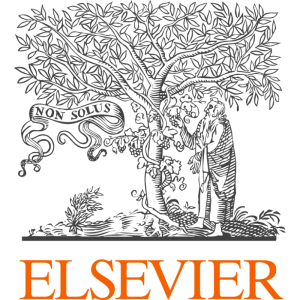
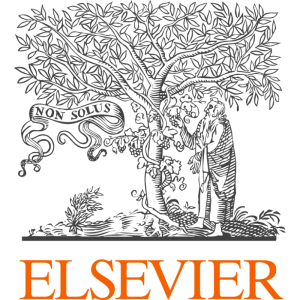
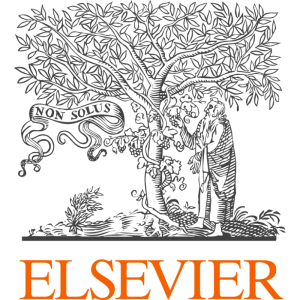
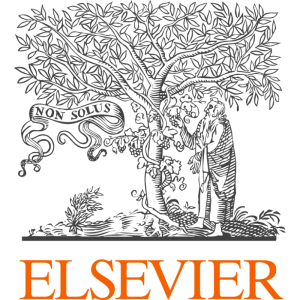
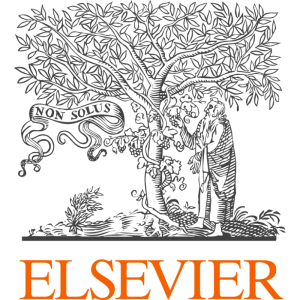
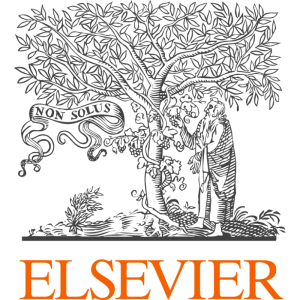
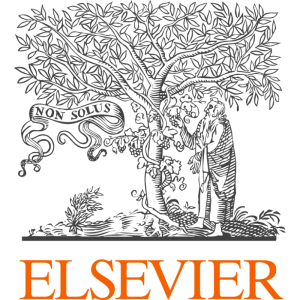
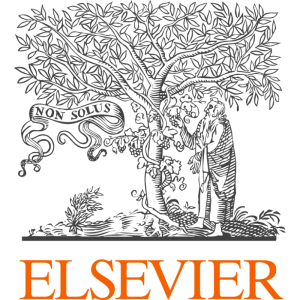
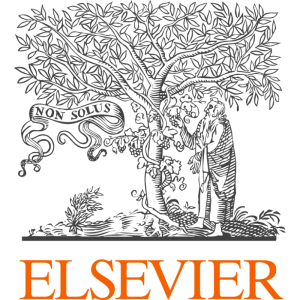
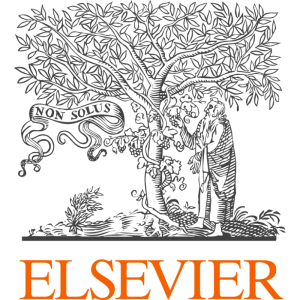
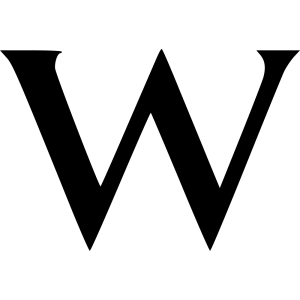
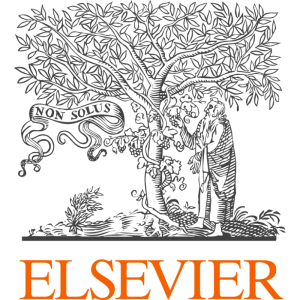
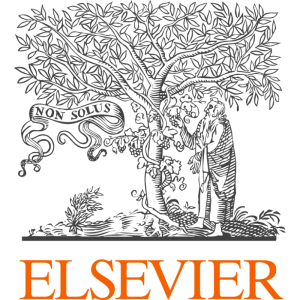
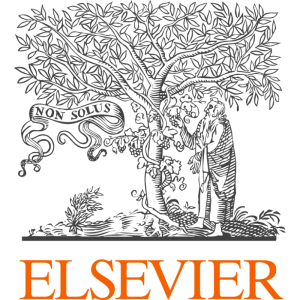
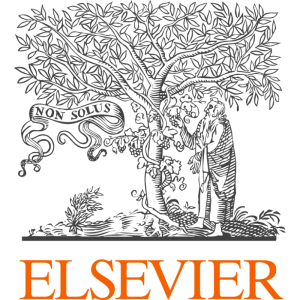
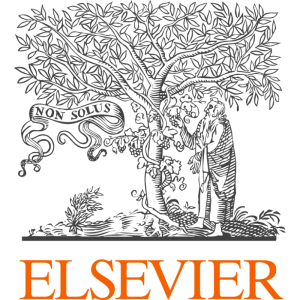
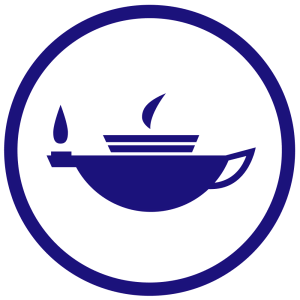
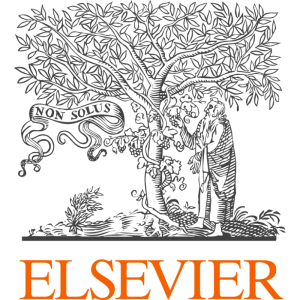
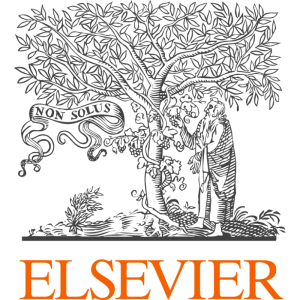
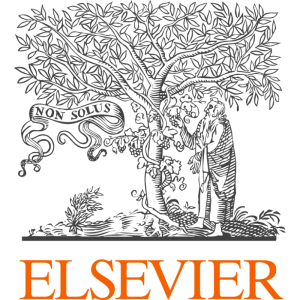
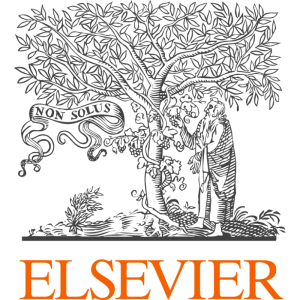
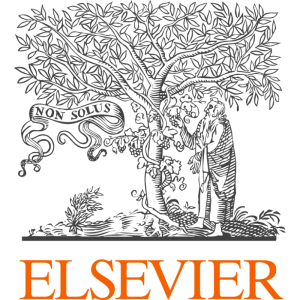
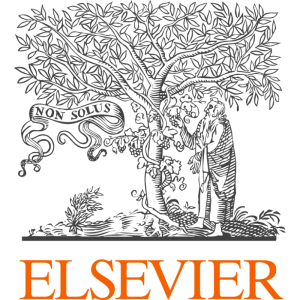
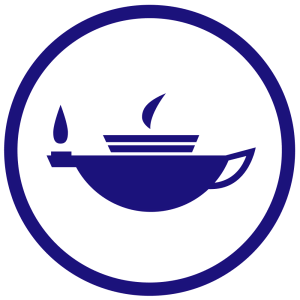
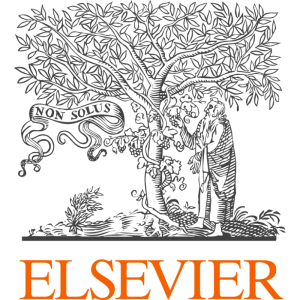
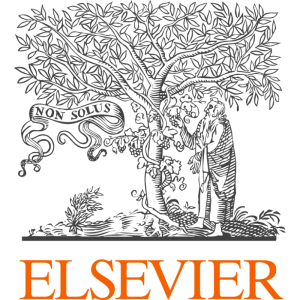
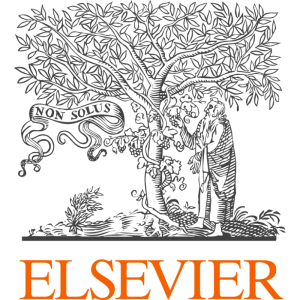
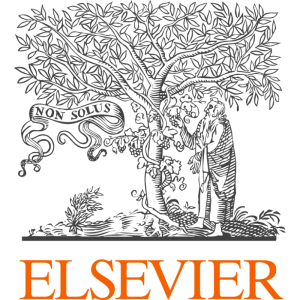
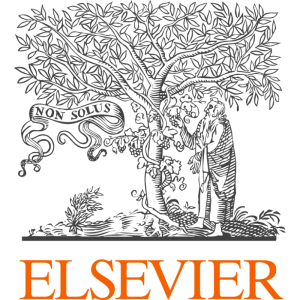
![Molecular interactions in N-[(2-hydroxyl)-propyl-3-trimethyl ammonium] chitosan chloride-sodium alginate polyelectrolyte complexes](/storage/images/resized/GDnYOu1UpMMfMMRV6Aqle4H0YLLsraeD9IP9qScG_small_thumb.webp)
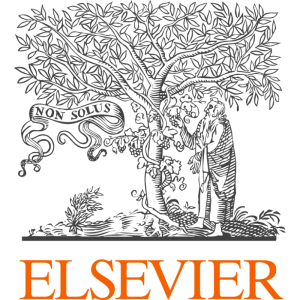
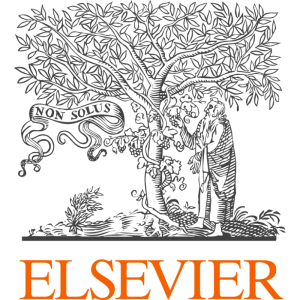
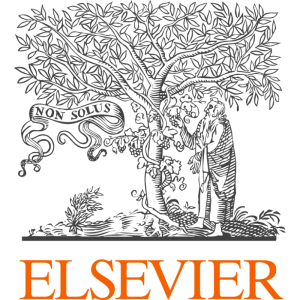
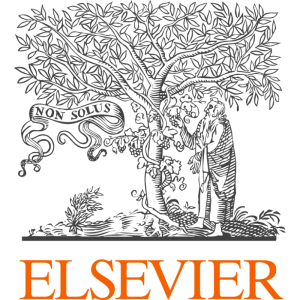
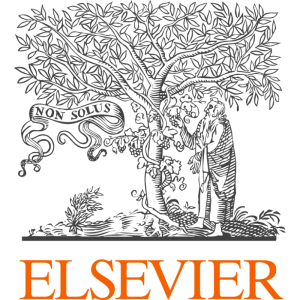
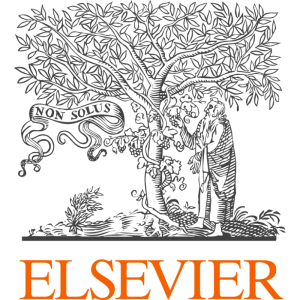
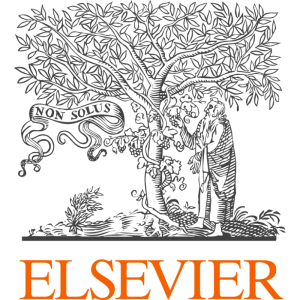
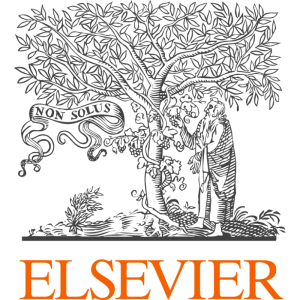
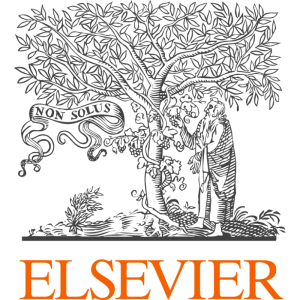
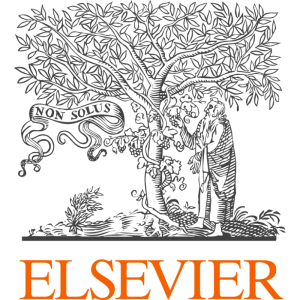
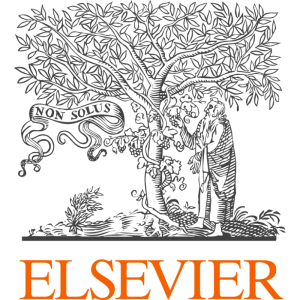
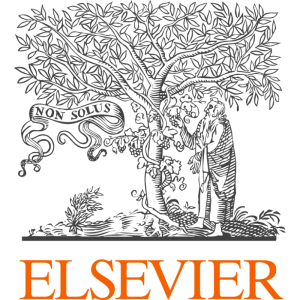
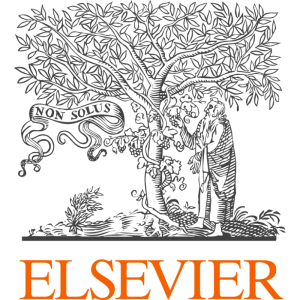
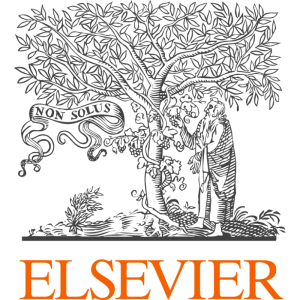
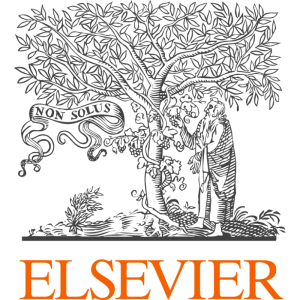
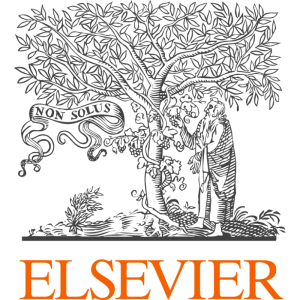
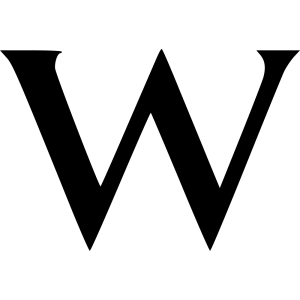
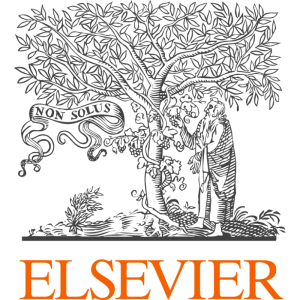
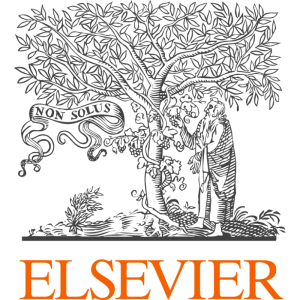
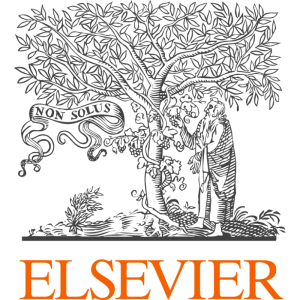
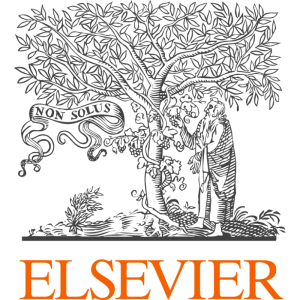