Keywords
Abstract
Data on the mechanochemical synthesis of halogen-containing organic compounds published mainly over the past 10 years are summarized and critically analyzed. The established views about the methods of activation of mechanochemical halogenation reactions are discussed. The mechanochemical fluorination, chlorination, bromination and iodination reactions of organic compounds are considered. Particular attention is paid to reaction mechanisms, selectivity problem and consideration of the mechanochemical approach as a promising trend of green chemistry. The review is devoted to applications of mechanochemistry in organic synthesis, while the fundamentals of mechanochemistry are beyond the scope of this publication.
The bibliography includes 155 references.
1. Introduction
Organohalogen derivatives are an important class of organic compounds that find practical use as drugs[1-12], chemosensors[13], luminophores[14], photosensitive and luminescent materials[15, 16], agrochemicals[17], polymers[18, 19], agents for diagnosis of various diseases[10], etc. Approximately 30% of new medicinal agents contain a fluorine atom[10]. It was shown that elemental chlorine is involved in the synthesis of 50% of industrial compounds 30% of agrochemicals and 20% of pharmaceuticals[20]. Some halogen-containing compounds are effective antibiotics, antiviral or anticancer agents, and function as signalling molecules. For example, trovafloxacin, ciprofloxacin, chloramphenicol, enteroseptol and tebrofen are antibacterial, antimicrobial and antiviral drugs with a broad range of action, while some organoiodine compounds, for example, thyroxine and triiodothyronine, are highly important human hormones (Fig. 1)[10, 21-24]. Chlorine, bromine and iodine are present in many pharmaceutical agents[21]. In medicinal chemistry, the introduction of a fluorine atom into the molecule increases the metabolic stability of drugs, often improving their transport[10], while the presence of chlorine enhances binding to molecular targets[25]. The introduction of fluorine into an aromatic ring usually increases the drug lipophilicity[26]. The presence of a fluorine atom in a pharmaceutical agent may sharply affect its metabolism[10, 26]. One more aspect of the fluorine chemistry is that fluorinated aromatic reagents can be used for modification of important biomolecules. For example, fluorinated heteroaromatic reagents are successfully used to modify peptides and amino acids[27, 28]. 4'-Fluoronucleosides are considered to be promising antiviral agents[29]. The introduction of a 18F label into arenes and heteroarenes is used in the positron emission tomography for both clinical and preclinical trials[30-32].
Both conventional solvents (CH2Cl2[33], CCl4[34], PhCl[35], MeCN[10, 36-38], etc.) and non-conventional ones (ionic liquids or their mixtures with organic solvents[39-44] and water[45, 46].) are widely used for halogenation of organic compounds. Green chemistry and its twelve principles[47-49] require the use of environmentally acceptable solvents and reagents and design for energy efficiency. Since the amount of solvents used for halogenation is usually rather large, this has an adverse effect on some green chemistry characteristics, resulting in larger amounts of waste, toxicity, increase in the mass balance (E-factor) and low energy efficiency. According to Sheldon’s statement[50], ‘the best solvent is no solvent’. Most of conventional solvents cannot be regarded as environmentally acceptable, and the reactions in these solvents are often non-selective. In recent years, attention has been drawn by mechanochemistry, an area of green chemistry in which the use of solvents is excluded or minimized and which may increase the selectivity of halogenation. According to the IUPAC classification, mechanochemistry has been regarded as an innovative method[51]. It is not surprising that quite a number of recent reviews are devoted to the use of mechanochemistry in the synthesis of organic compounds[52-63]. However, data on the direct mechanochemical halogenation included in these reviews are very scarce and are usually limited to a few examples. In most publications devoted to the synthesis of halogen organic compounds, halogens are first added to the substrate and/or the reagent, and then the mechanochemical synthesis is carried out. This synthesis includes diverse organic reactions: formation of heterocyclic compounds[64, 65], Suzuki–Miyaura cross-coupling[66]; arylselenation[67]; catalytic reactions of sulfonimidamides with ketones[68, 69]; cycloaddition of coumarin hydrazides to aromatic aldehydes in the presence of I2[70]; catalytic reactions of trifluoromethylarenes with nitroarenes[71]; condensation of tranexamic acid with arylaldehydes[72]; condensation of arylalkynes with aryl bromo ketones and NaN3[73]; N-formylation of arylamines[74]; reactions of amines with hydroxyiminoketones and aldehydes[75], fluorinated aromatic aldehydes[76] and esters;[77] cross-coupling[78] and reactions of benzoic acids with arylalkynes[79]. In our opinion, direct mechanochemical halogenation of organic compounds conducted as a green chemistry process is of most interest for organic chemists. The purpose of this review is to integrate and critically analyze the achievements in this field reported mainly in the last decade. Earlier publications are cited only to an extent to which they are necessary for the understanding of the essence of the problem and for identification of new trends in this field.
2. Methods of mechanochemical activation
Mechanochemistry includes chemical methods that utilize mechanical energy such as compression, shear or friction as a driving force for a chemical reaction. The energy can be supplied in different ways, e.g., by grinding reactants in a mortar, collision of the milling balls in the ball mills, or by shear of reactants in extruders. The history of the development of mechanochemical synthesis is described in recent reviews[80-83]. The origin of “unconscious’ mechanochemistry dates back to the prehistoric times, but wide use of this method in organic chemistry, including halogenation reactions, started only in the 1960s. The increased interest in mechanochemistry is indicated by the SciFinder data: the search for the term ‘mechanochemical’ gives back more than seven thousand references only over the last decade. Initially, researchers conducted reactions using simple mechanochemistry methods such as grinding of the substrate and the reagent in a mortar with a pestle. A benefit of this method is the simplicity and the possibility of using small amounts of compounds. However, this method does not always provide a good reproducibility of the results and depends on the human operator. Currently, the following mechanical tools are used most often in the mechanochemistry: vertical vibrational minimill, vibratory micro-mill, vibratory ball mill, planetary ball mill, twin-screw extruders, etc[84-88]. Halogenation is performed, most often, in ball mills in which the collisions of the milling balls supply energy to the reactants. In this case, the milling equipment is thoroughly selected considering the chemical and mechanical strength of the balls. It is also important that the material of the mill should be sufficiently hard and should not exhibit a catalytic activity towards halogenation. Materials commonly applied for mechanical grinding such as steel, ZrO2, SiO2 and WC can be regarded as acceptable for performing mechanochemical halogenation. Other materials such as Cu, Ni, Pt and Au are relatively soft and can exhibit catalytic activity. The use of ball mills provides considerable benefits as regards simplicity, selectivity and the yield of products[89]. The number, weight, size or material of the balls and the rotation velocity of the grinding part of the mill are important variables determining the result of mechanochemical halogenation. Mechanochemical reactions can also performed without milling bodies using the resonant acoustic mixing (RAM) technique[90]. In this method, the container is set into the oscillating motion at a fixed resonance frequency (usually approximately 61 Hz). In this case, the intensity of mixing is adjusted by varying the oscillation amplitude. Whereas in a usual ball mill, the physical impact can damage the solid particles and often causes aggregation of the reagents and substrates, the RAM technique minimizes the particle damage. Therefore, RAM is more appropriate for mixing reagents and substrates that are prone to stick together.
Most mechanochemical reactions are carried out using solid reagents and substrates without a solvent, although the addition of a small amount of liquid phase often accelerates the reaction and increases its selectivity[63, 89]. Since the isolation of a final reaction product is associated with energy consumption for solvent heating, pumping or cooling, the use of solvents is a major cause of waste accumulation and decrease in the energy efficiency in organic chemistry[91]. Moreover, if the solubility of the substrate in the corresponding solvent is low, the list of solvents greatly increases. Technically, mechanochemical methods are applicable to different states of halogenation reagents including gases, liquids and solids, while the catalyst and the substrate are usually in the solid state.
The application of mechanochemical halogenation methods is meant to pursue the following goals:
(1) preparation of products using atom-economic reactions without a solvent or with a minimum volume of a solvent,
(2) production using non-hazardous halogenation reagents,
(3) application of economical sources of mechanical energy,
(4) use of catalysts and multicomponent reactions to prepare complex halogenated compounds[52, 92].
3. Halogenation reagents
Molecular fluorine (F2) is an electrophilic fluorinating reagent well-known in organic chemistry[93, 94]. Although F2 meets the atom-economy principle, it is fairly toxic and harsh, requiring special equipment for fluorination. Selective fluorination of organic compounds cannot be performed even at low temperature and in the presence of acids. Fluorination with molecular fluorine is highly exothermic; hence, safety is of paramount importance for these reactions. This problem is partly solved by using low temperature, dilution of fluorine with an inert gas (Ar or N2) and by using flow reactors. When flow reactors are used, the amount of F2 in the reaction zone is low, which increases safety of the process[95].
In the last 50 to 60 years, oxygen-containing fluorinating agents (AcOF, CF3OF, FClO3, CsSO4F) and xenon difluoride have been widely used[10, 94, 96]. However, most of oxygencontaining fluorinating reagents are toxic and insufficiently stable, and F2 is often required to obtain these reagents in situ. Xenon difluoride is a potent fluorinating agent. It is more convenient for handling than F2 or OF reagents, as it is a crystalline solid that can be used for experiments in air; however, it is fairly toxic and expensive. Like fluorine, xenon difluoride is a strong oxidant, which makes it difficult to attain a high selectivity in the fluorination of organic compounds and often leads to the formation of by-products. An important achievement of the organofluorine chemistry in the last 40 years is the use of N–F reagents, which started to be actively employed in the electrophilic fluorination of organic compounds[10, 94, 97-99]. The N–F reagents are crystalline solids and are superior to О–F reagents in the stability, environmental friendliness and safety of handling. The major drawbacks of these compounds are the use of molecular fluorine for their synthesis and relatively low content of active fluorine (low Е factor). The F reagents that have been widely used to introduce a fluorine atom by the mechanochemical method include 1-chloromethyl-4-fluoro-1,4-diazoniabicyclo[2.2.2]octane bis(tetrafluoroborate) (Selectfluor, FTEDA-BF4, 9), N-fluorobis(phenylsulfonyl)amine (NFSI, 10), N-fluoropyridinium tetrafluoroborate (NF-Py, 11) and fluoroiodane (12) (Fig. 2). Selectfluor 9 and NFSI 10 are in most demand. The mechanochemical chlorination, bromination and iodination were performed using N-halosuccinimides [halogen = Cl (13), Br (14) and I (15)], 2,4,6-trichlorocyclohexane-1,3,5-trione (16), 2,4,6-tribromocyclohexane-1,3,5-trione (17), which are commercially available and fairly convenient for handling. The reagents such as KICl2, Cl2, NaBr and I2 are usually utilized in combination with oxidants.
4. Mechanochemical fluorination
The introduction of a fluorine atom into organic molecules is highly important for organic chemistry. For many years, direct fluorination was considered to be the most appropriate method for introduction of fluorine into organic compounds.
4.1. Transformation of the С(sp3)–H bond into the С(sp3)–F bond
Mechanochemiscal methods enable the C(sp3)–H to C(sp3)–F bond transformation in enolizable substrates on treatment with electrophilic fluorinating reagents.
In 2017, Browne and co-workers[100] reported the selective mechanochemical fluorination of 1,3-dicarbonyl compounds 18 under the action of the Selectfluor reagent 9 (Scheme 1).
The authors used a vibratory ball mill with a 10-mL milling jar and one stainless-steel ball of 10 mm in diameter. In the fluorination of 1,3-diphenylpropane-1,3-dione, the use of a minor amount of MeCN additive (0.13 mL g–1 relative to the total weight of the reactants) made it possible to predominantly obtain the monofluoronated product 19 (R1 = R2 = Ph), while the addition of 1.0 equiv. of Na2CO3 resulted in the difluorinated compound 20 (R1 = R2 = Ph) as the major product. The addition of other solvents (toluene, CH2Cl2, PriOH) also led to the predominant formation of monofluorinated product 19, although in a lower yield (9–30%). Notably, the addition of water completely inhibited the fluorination (the product yield was 0%). Unfortunately, the authors did not give any explanation for this fact, although they noted that the effect of additives on the selectivity of the mechanochemical process is difficult to substantiate. The effects of MeCN and Na2CO3 on the mechanochemical fluorination of other 1,3-diketone derivatives were similar to that observed for 1,3-diphenylpropane-1,3-dione (Table 1). A more pronounced effect brought about by the mechanochemical method in comparison with the fluorination in a MeCN solution was a decrease in the reaction time. The replacement of a mixer mill by a twin-screw extruder made it possible to scale up the mechanochemical fluorination of 1,3-dicarbonyl compounds, while retaining high selectivity[101, 102].
The addition of a minor amount of acetonitrile for the mechanochemical fluorination of β-ketoesters 21 in a ball mill is also crucial for increasing the reaction rate and increasing the selectivity to the monofluorinated product 22 (Scheme 2). It is noteworthy that the use of NaCl as an additive decreases the total yield of fluorinated products from 70 to 32%, although this salt is often used to facilitate milling[103].
The difluorination of 3-oxo-3-phenylpropanonitrile (24) by mechanochemical grinding with 2 equivalents of Selectfluor 9 for 3 hours afforded 2,2-difluoro-3-oxo-3-phenylpropanonitrile (25) in 65% yield. A similar reaction in a MeCN solution was very slow, and after stirring at room temperature for 3 weeks, difluoride 25 was isolated in only 4% yield[103].
A method for highly enantioselective mechanochemical fluorination of β-ketoesters 26 with NFSI 10 catalyzed by the complex Cu(OTf)2 with ligand 27 (Scheme 3) has been developed. High yields and enantioselectivities were attained using a small amount of the catalyst (5 mol.%) and a short reaction time (4 min)[104]. An increase in the rotation frequency of the ball mill from 100 to 600 rpm had virtually no effect on the yield and selectivity of fluorination of ketoester 26 (R1 = H, R2 = Me).
The yield and fluorination selectivity of 1,3-dicarbonyl compounds 29 considerably depend on their structure (Scheme 4). Acyclic β-ketoesters 34 and 35 were converted to fluorinated products in lower yields and with lower enantioselectivity than cyclic derivatives 31 and 32; the authors attributed this difference to different rigidity of substrate molecules. In the case of compound 35, the reaction carried out in solution (СН2Cl2, Et2O, PhMe) resulted in considerably lower yields and lower enantioselectivity than the mechanochemical process (20–34%, 25–0% ee vs. 60% and 61% ee)[104].
The mechanochemical fluorination of pyrazoles 36, formed in situ from 1,3-dicarbonyl compounds 21 and arylhydrazines 37, with Selectfluor 9 in a mixer mill in the presence of Na2CO3 was fairly selective and gave products in moderate to good yields (Scheme 5)[105]. In relation to pyrazole 36 (R1 = R2 = H), it was shown that the addition of 1 equiv. of Na2CO3 increases the yield of difluoride 38 (R1 = R2 = H) in the mechanochemical fluorination from 83 to 100%.
A characteristic feature of mechanochemical synthesis without a solvent is too low rate of diffusion of the reactants towards each other. At high-speed mixing, a local temperature rise at the site of impact may induce melting of the reactants, which should accelerate the reaction. However, the intense impact can also induce decomposition of organic compounds. The addition of a small amount of solvent (l-grinding) usually accelerates the mechanochemical transformation. A possible mechanism of this effect is the formation of a thin interfacial layer at grain boundaries of solid reactants or a solution of both reactants at the interface. A minor amount of the [Emim]NTf2 ionic liquid added to the system increases the rate and the product yield of mechanochemical fluorination of 2-methylcyclopentane-1,3-dione (39) and phenylbutazone (40) with Selectfluor 9 (Scheme 6)[106]. The relatively low yield of product 42 is probably due to sticking together of the substrate and the reagent during fluorination. Apparently, sticking could be avoided by using the RAM technique mentioned above.
The use of the Ag2O/TiO2 catalyst prepared by a mechanochemical process enabled fluorination via decarboxylation of carboxylic acids 43 in water to give fluorides 44 (Scheme 7)[107].
Hong and co-workers[108] proposed a method for mechanochemical fluorination of saturated compounds 45 with inactive C(sp3)–H bond using N-fluorobis(phenylsulfonyl) amine and addition of small amounts of EtOAc. Fluorinated products 46 were formed in moderate to high yields (Scheme 8)[108]. The authors suggested that fluorination occurs by a radical mechanism with the formation of imidyl radical via N–F bond cleavage. This is evidenced by the fact that the addition of radical traps such as 2,2,6,6-tetramethylpiperidin-Noxyl (TEMPO) or 2,6-di(tert-butyl)-4-methylphenol (BHT) to the reaction mixture inhibits fluorination of cyclohexane. A ball mill made of stainless steel containing Fe (~85%), Cr (12–14%) and Ni (0.6%) was used in the study. Note that ball mills made of nonmetals proved to be ineffective. Study of the effect of Cr, Fe or Ni metal additives on the fluorination of cyclohexane in SiO2 or ZrO2 jars showed that the addition of Cr or Ni does not lead to the formation of fluorocyclohexane, while the addition of Fe gives fluorocyclohexane in 4 and 11% yields[108].
4.2. Transformation of the C(sp2)–H bond into the C(sp2)–F or C(sp3)–F bond
The C(sp2)–H to C(sp2)–F bond transformation is usually accomplished by treatment with electrophilic fluorinating reagents. In search of versatile reagents compatible with mechanochemical methods, Geneste et al.[109] studied the reactivity of a number of arenes towards N-fluorobis(phenylsulfonyl)amine using ball milling in a 2-mL Eppendorf tube with four ZrO2 milling balls (total weight of 350 mg) at a frequency of 25–30 Hz[109]. Fluorination of methoxy-substituted benzene derivatives 58 and 62 was nonselective, giving both mono- (59, 60, 63) and difluorinated products 61, 64, 65 (Scheme 9).
The change in the mechanical milling frequency from 25 Hz to 30 Hz for the fluorination of 1,3,5-trimethoxybenzene 62 with NFSI 10 (1 equiv.) and the addition of SiO2 virtually did not affect the isomer ratio of the products, while the use of two equivalents of NFSI furnished mainly difluorinated product, that is, 4,4-difluoro-3,5-dimethoxy-2,5-cyclohexadienone 65. Other substrates such as anisole, N-benzylaniline and naphthalene did not react with NFSI under analogous conditions. However, 2-naphthol, which is a more reactive arene, underwent difluorination to give 1,1-difluoronaphthalen-2(1H)-one as the major product, the yield of which increased as the amount of NFSI increased from 1 equiv. (29%) to 2 equiv. (51%).
An efficient mechanochemical method for the fluorination of naphthols 66 with Selectfluor 9 has been proposed. In this method, products 67 and 68 were isolated by sublimation without a solvent (dry method) (Scheme 10)[110]. The solid-phase nature of the reactions was confirmed by scanning electron microscopy in relation to 2-naphthol 66 (X = Y = H).
The fluorination of estrone 69 by simple grinding of the substrate and the reagent in a mortar afforded a mixture of three fluorination products 70–72 (Scheme 11).
The effect of small amounts of various ionic liquids (73–76) on the rate and selectivity of the mechanochemical fluorination of 2-naphthol (66) in a vibratory mortar grinder with a moving ball has been studied (Scheme 12)[106]. The fluorination conversion varied from 16 to 72%, and the 67:68 ratio was in the range from 0.5 to 3.9. Ionic liquids with TfO– and Tf2N anions and low-viscosity ionic liquids proved to be the most efficient catalysts.
The mechanochemical fluorination of naproxen 77 with Selectfluor 9 afforded 2-(5-fluoro-6-methoxynaphthalen-2-yl)- propionic (78) and 2-(5,5-difluoro-6-oxo-5,6-dihydronaphthalen-2-yl)propionic (79) acids as the major products (Scheme 13)[106, 111]. The same products were obtained by fluorination of naproxen in MeCN and H2O, where it was shown that water served as the source of the oxygen atom for difluoride 79[45]. Presumably, in the case of mechanochemical fluorination of naproxen, humid air was the source of oxygen.
The mechanochemical Mannich reaction of ketimines 80 with pyrazolones 81 in combination with chiral catalyst 82 followed by fluorination with NFSI 10 in the presence of minor amounts of CH2Cl2 gave fluorination products 84 in high yields (66–98%) with a high enantiomericic ratio (er) of up to 99:1 (Scheme 14)[112]. The authors used a vibratory ball mill, in which milling jars with stainless-steel balls made radial oscillations with frequencies from 3 to 30 Hz. The predominant formation of the (3S,4R)-diastereoisomer 84 (R1 = R2 = H) was confirmed by B3LYP-D3/6-31G* calculations. According to the calculations, the transition state leading to the (3S,4R)-diastereoisomer is 50.8 kJ mol–1 more favourable than that leading to the (3S,4S)- diastereoisomer; this is partly due to stabilization of the transition state for the major (3S,4R)-diastereoisomer by three hydrogen bonds.
The mechanochemical fluorocyclization of hydrazones 85 and oximes 86 on treatment with fluoroiodane reagent 12 with (CF3)2CHOH and CH2Cl2 additives afforded tetrahydropyridazines 87 and dihydrooxazines 88, respectively, in high yields (Scheme 15)[113]. The authors used a stainless-steel vibratory mill (10 mL) with 2.5-g milling balls; the reaction mixture was milled at a frequency of 30 Hz for a certain time period (15–60 min). It was shown that mechanochemical fluorination of oximes gives fluorinated products in higher yields (74–80%) than fluorination in CH2Cl2 solutions (42–53%).
The authors believe that the reaction of fluoroiodane 12 with oxime 86 affords π-complex А, which subsequently undergoes cyclization (Scheme 16).
5. Mechanochemical chlorination
5.1. Transformation of the C(sp3)–H bond into the C(sp3)–Cl bond
The C(sp3)–H to C(sp3)–Cl bond transformation is performed using electrophilic chlorinating reagents and readily enolizable substrates.
Moorthy and co-workers [114] reported the mechanochemical chlorination of crystalline β-keto dicarbonyl compounds 18 with trichloroisocyanuric acid 16 (Scheme 17). The authors used a ball mill with a 5-mL stainless-steel milling jar containing 3-mm stainless-steel balls. It was noted that the use of trichloroisocyanuric acid gives an atom-economy effect. Even when the acid to substrate molar ratio was 0.7, dichlorides 89 (X = Cl) were formed in all cases in high yields (95–97%). A change in the oscillation frequency of the mill from 5 to 15 Hz and a change in the number of balls from 5 to 15 virtually did not affect the yield of products.
Monoketones proved to be less reactive. However, the use of 25 mol.% p-toluenesulfonic acids (90) as a catalyst made it possible to perform the mechanochemical chlorination of substituted acetophenones 4-RC6H4COMe (91: R = Br, NO2), 1-indenone (92) and 5,7-dibromo-3,4-dihydronaphthalen-1-one (93) with reagent 16 at room temperature to give chloro derivatives 94–96 in 90–94% yields[114].
Grynszpan and co-workers 115 carried out mechanochemical chlorination of 3,4-dimethyl-2-pyrazolin-5-one (97) with trichloroisocyanuric acid (16) to give chlorinated product 98 (69%) (Scheme 18)[115]. The authors used simple grinding of the reagent and the substrate in a mortar with a pestle. The choice of trichloroisocyanuric acid as the chlorinating reagent provides atom economy, because chlorination requires only 1/3 equivalents of the reagent. In the authors’ opinion, chlorination proceeds via the formation of enol form 99.
Chloroketones 100, prepared by the mechanochemical chlorination of ketones 101 with trichloroisocyanuric acid (16) in the presence of p-toluenesulfonic acid (90), were subsequently used for the condensation with thiourea (102) to obtain 2-aminothiazoles 103 (Scheme 19)[116].
The use of CeO2 or Al2O3 catalyst in the mechanochemical chlorination of methane with trichloroisocyanuric acid (16) made it possible to reduce the reaction temperature (90 vs. 200°C) and to sharply improve the selectivity to CH3Cl (95 vs. 66%) at 30% convesion[117]. Among catalysts used for methane chlorination with this reagent (MgO, Fe2O3, SiO2, Al2O3, TiO2, CeO2), the highest activity was found for CeO2[118]. The authors of the patent [118] noted that N-chlorosuccinimide (NCS) 13 can be used as the halogenation reagent, apart from trichloroisocyanuric acid 16, and hydrocarbons containing 1 to 8 carbon atoms in the molecule are applicable chlorination substrates.
5.2. Transformation of the C(sp2)–H bond to the C(sp2)–Cl bond
Aryl chlorides 104 (X = Cl) are formed from arenes 105 under the action of NaCl and KHSO5 as the oxidant[119]. In the case of monosubstituted benzenes, the chlorine atom enters mainly the ortho- and para-positions relative to the electron-donating substituent, which attests to the electrophilic nature of the reaction (Scheme 20). Benzene and substituted benzene derivatives RC6H5 with electron-withdrawing substituents (R = Cl, CN, NO2) are not chlorinated. It is noteworthy that chlorination is often non-regioselective and occurs with high conversion (the conversion is given in parentheses).
As opposed to this, the use of NCS 13 and the (NH4)2[Ce(NO3)6] oxidant results in the regioselective mechanochemical chlorination of mono- and bicyclic aromatic compounds 105. The high yields of monochlorinated products 118, 120–122 (82–92%) attest to good selectivity of the reaction (Scheme 21)[120].
A mechanical mortar–pestle was used for the regioselective mechanochemical chlorination of phenols 104 (X = OH) and 2-aminopyridine with NCS (13) in the presence of a small amount of poly(ethylene glycol) (PEG-400) (Scheme 22)[121]. In the synthesis of products 124 and 125, the H2SO4 additive (10 mol.%) was used. The short reaction time is probably due to the fact that PEG-400 increases the polarization of the N–X bond in NCS, and the Cl+ ion is formed faster. In addition high concentration of the substrate and the reagent may be generated in the PEG-400 layer upon grinding, which could also accelerate the reaction. The high regioselectivity is apparently caused by the formation of the thermodynamically more stable σ-complex.
Trihaloisocyanuric acids (THICA) are efficient reagents for the direct halogenation of electron-rich aromatic compounds using a ball mill[114]. The presence of three halogen atoms in THICA make them highly advantageous for attaining atomeconomy in the halogenation. Moorthy and co-workers[114] used trichloroisocyanuric acid (16) for the mechanochemical chlorination of aromatic and heteroaromatic compounds 105 to give chlorides 127–135 in moderate to high yields (Scheme 23). The reactions were carried out using a ball mill in a 5-mL stainless-steel jar containing 3.0 mm balls also made of stainless steel, without any protection from the atmosphere. Trichloroisocyanuric acid 16 is a source of three chlorine atoms; therefore, even when the acid to substrate molar ratio was 0.7, dichlorides were formed in all cases. For the synthesis of tetrachloride 135, a 1,4-fold excess of acid 16 over the substrate was used.
Barišić et al.[122] performed the mechanochemical chlorination of substituted azobenzenes 136 with NCS (13) in an IST500 mixer mill. The reactions were carried out in a 14-mL poly(methyl methacrylate) jar and tungsten carbide milling balls with a diameter of 7 mm with addition of silica gel to improve grinding. The reaction proceeded fairly selectively to give chloroazobenzenes 137 (X = Cl) in 46–85% yields. Chlorination involved predominantly the phenyl moiety with an electrondonating substituent, which is indicative of the electrophilic nature of the reaction (Scheme 24). The lowest yield (46%) was observed for compound 137 with the electron-withdrawing NO2 group (R1 = NO2, R2 = NMe2).
The use of NCS (13) as the reagent for the cascade formation of the C–N bond from biphenyl-2-carboxamides 138 followed by mechanochemical chlorination resulted in the formation of phenanthridinones 139 in high yields (85–94%) (Scheme 25)[123]. The mechanochemical experiments were carried out in an MM 200 mixer mill (operating frequency of up to 25 Hz) in a 10-mL jar with one stainless-steel milling ball of 15 mm in diameter. The authors noted that chlorination with reagent 13 is mainly directed into the para-position relative to the formed C–N bond of phenanthridinone and is inhibited when this position is occupied by a substituent.
The regioselective mechanochemical chlorination of imidazo[1,2-a]pyridines 140 was performed by mere grinding in a mortar with NaCl or NH4Cl and (diacetoxyiodo)benzene (141), which served as the oxidant (Scheme 26)[124]. The yield of chlorinated derivatives 142 (X = Cl) was 55–60%.
When a mixture of CuCl2 (95 mass%) and biphenyl or naphthalene (5 mass%) was milled at a temperature of 80–170 °С, chlorination occurs non-selectively to give a set of products containing 1 to 10 and 1 to 8 chlorine atoms, respectively[125]. The mechanochemical synthesis was carried out using a TB-1 high-energy planetary ball mill at 890 rpm in a stainless-steel jar (0.3 L) with a ceramic ball of 10 mm in diameter.
The mechanochemical chlorination of nickel porphyrin complexes 143 with a 14-fold excess of NCS (13) in a ball mill proceeded fairly selectively to give chloroporphyrin complexes 144 (Scheme 27)[126]. The use of a jar and balls made of steel instead of SiO2 and a decrease in the ball diameter from 10 to 5 mm resulted in a decrease in the yield of product 144 (R = Н) down to zero instead of 62%. Obviously, the material hardness and the size of milling balls affect the kinetic energy at collisions, which is essential for the mechanochemical chlorination of nickel porphyrin complexes.
5.3. Transformation of the X–H bond into the X–Cl bond (X = N, S)
Emmerling and co-workers[127] investigated the kinetics of chlorination of N-3-ethyl-5,5-dimethylhydantoin (145) with Ca(ClO)2 to give monochlorinated derivatives 146 using a ball mill with different diameters of the balls (2, 4, 5, 6 and 8 mm) (Scheme 28). The kinetic data indicated that the course of the reaction up to the equilibrium was the same for different sizes of the milling balls.
Upon the oxidative chlorination with NCS (13) or 1,3-dichloro-5,5-dimethylimidazoline-2,4-dione (147) in a mixer mill, sulfinamides 148 were quantitatively converted to sulfonimidoyl chlorides 149 (Scheme 29). When trichloroisocyanuric acid (16) was used, the yield of the chlorinated product was lower (56%)[128]. The reactions were carried out in a mixer mill with a 12-mL ZrO2 jar containing eight 3-mm balls of the same material at a frequency of 25 Hz.
6. Mechanochemical bromination
6.1. Transformation of the С(sp3)–H bond into the С(sp3)–Br bond
Like in the case of fluorination and chlorination, in the electrophilic bromination, C(sp3)–H to C(sp3)–Br bond transformation occurs most smoothly for easily enolizable substrates. Moorthy and co-workers [114] performed the mechanochemical bromination of ketones 18 with tribromisocyanuric acid (17), which gave dibromides 89 (Х = Br) in high yields (see Scheme 17). Owing to the presence of three bromine atoms in tribromoisocyanuric acid 17, it was used in 0.7:1 molar ratio to the substrate, which complies with the atom economy principle.
Wang and Gao[129] carried out the mechanochemical bromination of 1,3-dicarbonyl compounds 18 using NaBr (2–2.5 equiv.) and KHSO5 (1 equiv.) as an oxidant (Scheme 30). The reactions were carried out in a Retsch MM200 ball mill using a stainless-steel jar (5 mL) with steel balls of 7 mm in diameter. Acyclic dicarbonyl compounds were mainly converted to monobromides 150, while cyclic compounds gave dibromides 87. This difference can be attributed to higher reactivity of cyclic 1,3-dicarbonyl compounds.
6.2. Transformation of the С(sp2)–H bond into the С(sp2)–Br bond
The use of a ball mill and NaBr as the reagent in combination with the KHSO5 oxidant enabled direct bromination of a broad range of arenes 105 to give the corresponding bromo derivatives 104 (X = Br) (see Scheme 20)[119]. The selectivity of bromination was close to that for chlorination. It was shown in relation to the bromination of mesitylene that the conversion increased as the mill oscillation frequency was increased from 5 to 30 Hz, or the mixing time was increased from 1 to 30 min and changed only slightly upon the addition of small amounts of solvents (H2O, EtOH, CHCl3, n-heptane). The highest selectivity (>91%) to bromomesitylene was attained by using additives of quartz sand, γ-Al2O3 (basic), SiO2, K-10 clay and NaBr, while the lowest selectivity was observed in the presence of γ-Al2O3 (neutral) (7%), γ-Al2O3 (acid) (66%) and Na2SO4 (47%).
Bose and Mal [120] developed a method for chemo- and regioselective mechanochemical bromination of a wide range of aromatic compounds 105 with N-bromosuccinimide (NBS, 14) using a ball mill to give mono- and dibromides 152–157 in high yields (Scheme 31). The predominant ortho- and para-direction of the bromination relative to the electron-donating substituent attests to the electrophilic nature of the reaction
Moorthy and co-workers[114] used tribromoisocyanuric acid (17) for the mechanochemical bromination of aromatic and heteroaromatic compounds 100 (see Scheme 23). The product yields obtained in the bromination of substrates 105 with tribromoisocyanuric acid 17 were usually higher than the yields in the chlorination with trichloroisocyanuric acid 16. Even the use of a deficient amount of tribromoisocyanuric acid relative to the substrate (0.35 to 0.7) did not prevent the introduction of two bromine atoms into the molecule.
A mechanical mortar–pestle was used for regioselective mechanochemical bromination of a broad range of phenols and amines 104 giving bromo derivatives 158 (Scheme 32)[121]. As in the case of chlorination with NCS 13, the addition of a small amount of poly(ethylene glycol) (PEG-400) makes it possible to perform bromination over a short period of time because of the formation of a thin layer of the liquid phase at the substrate–reagent interface.
Pesyan et al. [130] reported the bromination of 1,3,5-trimethoxybenzene 62 and 2-naphthol 66 on treatment with BrCN in the presence of AlCl3 by grinding the reactants and the catalyst in an agate mortar (Scheme 33). The bromination of these substrates was accompanied by cyanation, with products 104 and 159 being formed in an equimolar ratio.
The mechanochemical bromination of naphthalene (105) with brominating reagents 14 and 160–162 in the presence of the CBV-760 zeolite gave 1-bromonaphthalene 118 in moderate (57–80%) to high (90%) yields (Scheme 34)[131]. In the absence of the zeolite, the reaction is sharply retarded, the yield does not exceed 8%. Milling experiments were carried out using ZrO2 milling balls and a Teflon jar. The authors noted that the use of steel equipment is undesirable because of its interaction with the zeolite. In the presence of a twofold excess of brominating reagent 162, 1,4-dibromonaphthalene was obtained in a yield of 87%.
The mechanochemical bromination of azobenzenes 136 with N-bromosuccinimide 14 proceeds fairly selectively to give bromoazobenzenes 137 in 37–54% yield; the incorporation of the bromine atom into position 3 is obviously caused by the presence of the neighbouring electron-donating R2 group (see Scheme 24)[122]. It is of interest that the authors were unable to chlorinate less active substrate 136 (R1 = H, R2 = MeO), whereas bromination with NBS gave bromo derivative 137 (R1 = H, R2 = MeO) in 90% yield.
The mechanochemical bromination of azobenzene 136 (R1 = R2 = H) with NBS (14) in the presence of Pd(OAc)2 and p-TsOH 90 is directed to the ortho-position to the N=N group and gives 2-bromoazobenzene 163 (R1 = R2 = H) (Scheme 35)[132]. The reaction was carried out in a 14-mL poly(methyl methacrylate) jar with a tungsten carbide milling ball of 7 mm in diameter. p-Toluenesulfonic acid is necessary for the activation of NBS.
The mechanochemical conversion of sulfoximine 164 to bromosulfoximine 165 was studied by solid-state 13C NMR spectroscopy using cross-polarization magic angle spinning (CP-MAS) technique (Scheme 36)[133]. Mixing was carried out in a ball mill at 25 Hz frequency using a poly(methyl methacrylate) jar and zirconia balls, and then the 13C NMR spectrum of the solid mixture was recorded without additional treatment. The benefits of the method include the possibility of monitoring the substrate and reagent consumption and the product formation by conducting the reaction in the NMR rotor. However, the authors noted that, despite the relatively high centrifugal pressure arising from the rotation of the MAS rotor, the efficiency of mixing was insufficient for complete conversion of substrate 164, and the reaction proceeded more slowly than in the ball mill. The problem might be related to the too low reactivity of the substrate.
The oxidative mechanochemical bromination of imidazo[1,2-a]pyridines 140 by treatment with NaBr or NH4Br and (diacetoxyiodo)benzene (141) as the oxidant proceeds regioselectively and gives bromides 142 (79–84%) in higher yields compared to the yields of chlorides (55–60%) in a similar chlorination reaction (see Scheme 26)[123]. The authors believe that the most probable bromination mechanism includes the in situ formation of (acetoxybromoiodo)benzene 166 and complex В (Scheme 37).
It is of interest that bromination does not take place if K2S2O8 is used as the oxidant instead of (diacetoxyiodo)benzene 141[124].
Bera and Mal[123] used a ball mill to prepare bromo-substituted phenanthridinones 139 (X = Br) by treatment of N-methoxy-[1,1'-biphenyl]-2-carboxamides 138 with NBS 14 (see Scheme 25). As in the case of chlorination, the halogen atom enters the para-position relative to the NOMe group of phenanthridinone 139. The use of ВНТ and TEMPO radical traps showed that bromination proceeds, in all probability, by a radical mechanism (Scheme 38)[123].
The dimer [Cp*RhCl2]2 (167) was used as a catalyst in the selective mechanochemical bromination of 2-phenylpyridine (168) with NBS 14 in the presence of AgSbF6 as an oxidant (Scheme 39)[134]. The reaction was carried out in a planetary mill at 800 rpm using a 12-mL ZrO2 jar with twenty ZrO2 balls of 5 mm in diameter.
To elucidate the mechanism of oxidative bromination, the authors synthesized complex 170 by treating 2-propylpyridine 168 with complex 167 in the presence of sodium acetate under the same conditions (see Scheme 39)[134]. It is noteworthy that the mechanochemical formation of this complex was faster than the reaction in CH2Cl2. Complex 170 was ground with NBS and AgSbF6; as a result, the major product 169 (X = Br) was obtained in 71% yield. These results indicate that complex 170 may be an intermediate of the catalytic bromination of 2-phenylpyridine in a ball mill.
6.3. Transformation of the C(sp2)–H bond into the C(sp3)–Br bond
Mal and co-workers[135] used a ball mill to prepare brominated oxazolines 171 by treating N-allylbenzamides 172 with N-bromosuccinimide 14 (Scheme 40). Even in the presence of electron-withdrawing substituents in the aromatic ring of N-allylbenzamide 172 (X = Cl, Br, I, NO2), products 171 (Y = Br) were formed in good yields (71–85%).
Wong and Yeung[136] developed an efficient method for mechanochemical bromo functionalization of olefins 173 using NBS 14 (Scheme 41). The reactions were carried out using a Retsch (MM 400) mixer mill in a 10-mL zirconia jar at ambient temperature away from light. In relation to olefin 173 (R = H, X = O), it was demonstrated that 1,3-dibromo-5,5-dimethyl hydantoin is applicable as a brominating agent, with the yield of product 174 (R = H, X = O) being 99%.
7. Mechanochemical iodination
7.1. Transformation of the С(sp2)–H bond into the С(sp3)–I bond
Aliphatic iodination is a challenging task in mechanochemistry because of high polarizability and low energy of the С–I bond and also low electrophilicity of the I+ cation. Mal and coworkers[135] prepared iodo oxazolines 171 (X = I) from N-allylbenzamides 172 via mechanochemical cascade cyclization and iodination with N-iodosuccinimide (NIS, 15), which gave rise to a C(sp3)–I bond in the final product (see Scheme 40)[135]. In the presence of TEMPO radical trap, the reaction afforded iodinated oxazoline 171 (X = H, Y = I) in a good yield (79%) under the usual reaction conditions, which is an argument against the radical reaction mechanism. The authors believe that the iodination follows an ionic mechanism and involves π-complex C (Scheme 40).
7.2. Transformation of the С(sp2)–H bond into the С(sp2)–I bond
Bose and Mal 120 developed a process for the regioselective iodination of reactive aromatic compounds 105 with NIS 15 using a ball mill (Scheme 43, conditions а)[120]. The main benefits of this process are mild conditions, short reaction time and high yields of iodination products.
The use of I2 in this mechanochemical reaction of aromatic compounds 105 required the addition of KHSO5 as an oxidant (see Scheme 43, conditions b)[137]. The solid reactants were introduced into a ball mill operating at a frequency of 21 Hz.
The iodination of p-toluidine 176 (R1 = NH2) with the monohydrate KICl2 ·H2O upon simple grinding of the reactants in a mortar with a pestle involves mainly the ortho-position relative to the amino groups, giving rise to mono- (177) and diiodinated products 178 (R1 = NH2) in 9% and 36% yields, respectively (Scheme 44)[138]. The replacement of the NH2 group in arene 176 by NAc resulted in the formation of only monoiodinated product 177 (R1 = NHAc) in a relatively low yield (5%).
Thiophene derivatives proved to be more reactive than aniline derivatives. For example, the mechanochemical iodination of 5-carbaldehyde-2,2'-bithiophene (179) under the same conditions resulted in the formation of product 180 in a quantitative yield (Scheme 45)[138]. When the reaction is carried out in water or CH2Cl2, the yield of product 180 is much lower (30 and 89%, respectively).
Wang and co-workers[139] developed a process for orthoiodination of acetanilides 181 catalyzed by Pd(OAc)2 using NIS 15 and an Spex SamplePrep 8000 mixer mill (Scheme 46).
The mechanism proposed for this reaction includes the formation of Pd(OTs)2 which is inserted between the ortho-С–Н bond of acetanilide and the С=О group, giving a PdIV complex (Scheme 47)[139].
The regioselective mechanochemical iodination of imidazo[1,2-a]pyridines 140 with NaI in the presence of an oxidant, (diacetoxyiodo)benzene (141), furnishes iodo derivatives 142 (X = I) in 70–78% yields (see Scheme 26)[124].
The mechanochemical iodination of 2-phenylpyridine (168) with N-iodosuccinimide 15 in the presence of the dimer [Cp*RhCl2]2 (167) and AgSbF6 as an oxidant is fairly selective, giving iodo derivative 169 (X = I) in a good yield (see Scheme 39)[134].
7.3. Transformation of the С(sp)–H bond into the С(sp2)–I or С(sp3)–I bond
The electrophilic cyclization of 2-alkynylanisoles 183 under mechanochemical conditions affords 3-iodobenzofurans 184, with an equimolar amount of iodine being sufficient to attain good yields (Scheme 48)[140]. The reaction was carried out in a ball mill with a 10 mL stainless-steel jar and one ball made of the same material of 10 mm in diameter.
The authors assumed that the reaction of molecular iodine with the 2-alkynylanisole triple bond results in the formation of π-complex D, which then undergoes cyclization (Scheme 49, path 1). Simultaneous iodo-alkynylation and demethylation (path 2) is also possible, which is more preferable in the absence of a solvent[140].
Sarkar and co-workers[141] proposed an efficient mechanochemical synthesis of polysubstituted quinoline derivatives (185) via oxidative cyclization of anilines 186 in air using simple mixing of the reactants with a pestle in a mortar (Scheme 50). Presumably, π-complex E is a key iodination intermediate.
Kolvari et al. 142 developed a facile and efficient procedure for the synthesis of iodoarenes 187–192 including the successive diazotization–iodination of aromatic amines 193 with sodium nitrite and potassium iodide in the presence of sulfonated particles supported on the nanomagnetic γ-Fe2O3 –SO3H material (Scheme 51).
Diazotization is usually accomplished by protonation of arylamines with nitrous acid in an acid medium (H2SO4, HCl) at a low temperature (0–10°С). Owing to the use of γ-Fe2O3 –SO3H and mechanochemical procedure, the process corresponds to green chemistry principles and can be conducted at room temperature[142].
8. Conclusion
Mechanochemical synthesis provides an environmentally acceptable alternative to approaches based on the chemistry of solutions. Elimination of organic solvents from a process reduces energy consumption and results in ‘greener’ processes. In recent years, mechanochemistry has become a widely used experimental method for selective and efficient introduction of halogen atoms into organic molecules. Many approaches to the preparation of halogenated organic compounds are based on the use of equimolar amounts of a substrate and a halogenating reagent, which increases the E-factor. Of particular interest are mechanochemical processes that exclude the use of solvents even for product purification, for example, by using sublimation (so-called, dry method)[110].
The mechanochemical fluorination of organic compounds is performed most often using NF reagents such as NFSI and Selectfluor. These reagents are commercially available and convenient for handling. Fairly accessible N-halosuccinimides, trihaloisocyanuric acids and I2 in combination with oxidants are widely used for chloro, bromo and iodo functionalization. The utilization of trihaloisocyanuric acids provides atom economy, because only 1/3 of the reagent molecule is enough to introduce a halogen atom.
The use of mechanical force to initiate a chemical reaction is an efficient alternative to traditional activation methods such as heat, light and electricity. In recent years, mechanochemical activation has been used in combination with above-mentioned energy sources. Mechanochemical processes performed at a controlled temperature or under light irradiation, acoustic treatment or electrical pulses make it possible to accomplish reactions that cannot be initiated by conventional mechanochemical treatment. Methods of this type, called thermomechanochemistry, sonomechanochemistry, electromechanochemistry and photomechanochemistry, are of considerable interest in modern mechanochemistry[143-146]. However, these methods have not yet found wide use in the synthesis of halogenated organic derivatives (cf. Refs [117, 118, 142]). Temperature variation upon mechanical treatment and cooling systems may facilitate the use of thermally sensitive substrates in reactions[147]. The application of mechanochemical methods in fundamental research laboratories and in industry is diverse, ranging from chemical synthesis in ball mills and ultrasonic baths to direct activation of covalent bonds using atomic force microscope[148]. Certain covalent bonds may be preactivated towards cleavage and substitution reactions, for example, for the replacement of hydrogen by a halogen atom.
The theoretical interpretation of mechanochemical reactions is a challenging task, because the mechanism of mechanical action is very specific. The mechanical properties of crystals are determined by their composition, interaction forces between components, structure and the presence of defects. Under the action of mechanical forces, solids demonstrate three types of characteristics: elasticity, plasticity and strength[149]. Regarding the understanding of mechanical properties, particular attention is paid to the macrokinetics and the role of mixing and milling types[150]. The regularities of mechanochemical transformations are determined by numerous parameters that markedly complicate conduction of processes under controlled conditions and prediction of the outcome[151]. The effect of mechanical force on single organic molecules is still obscure at a fundamental level, which limits the application of mechanochemistry. The use of solvents as additives in mechanochemical process (L-grinding) often provides a higher halogenation selectivity than the reaction in solutions. This is in line with the goals and objectives of green chemistry. However, the L-grinding method does not always explain the effect of solvents on the mechanochemical process, which requires further collection of experimental facts. Slow development of mechanochemical techniques for the synthesis of halogenated organic compounds is evidently attributable to the insufficient understanding of the prospects of this method. As a result, many chemists still resort to empirical rules when necessary.
Diffraction and spectroscopic methods make it possible to study the mechanochemical halogenation on a real-time basis, which opens up new opportunities for studying the reaction mechanism[133, 152, 153]. Understanding of mechanochemical processes at the molecular level can be greatly improved by using quantum chemistry and computer simulation[112, 154, 155]. The research of the use of mechanochemistry in the synthesis of halogenated organic compounds should be concentrated on the elucidation of the specific action of the medium on the reaction mechanism and on the factors determining the reaction rate and selectivity.
9. List of abbreviations and symbols
Atom economy is evaluated as the molecular mass of the final product divided by the total molecular masses of all reactants,
BHT — 2,6-di(tert-butyl)-4-methylphenol,
Bn — benzyl,
Boc — tert-butoxycarbonyl,
CBV-760 — zeolite,
Cy — cyclohexyl,
E-factor — amount of loss per kg of the product, including
solvents, catalysts and by-products
ee — enantiomeric excess,
er — enantiomeric ratio,
[Emim]NTf — 1-ethyl-3-methylimidazolium bis(trifluoromethylsulfonyl)imide,
IL — ionic liquid,
L-grinding — use of solvent additives in the mechanochemical
process,
NBS — N-bromosuccinimide,
NCS — N-chlorosuccinimide,
NF-Py — N-fluoropyridinium tetrafluoroborate,
NFSI — N-fluorobis(phenylsulfonyl)amine,
NIS — N-iodosuccinimide,
PEG-400 — poly(ethylene glycol) PEG-400,
RAM — resonant acoustic mixing,
Selectfluor — 1-chloromethyl-4-fluoro-1,4-diazoniabicyclo[2.2.2]octane bis(tetrafluoroborate),
TEMPO — 2,2,6,6-tetramethylpiperidin-N-oxyl,
Tf — triflyl (trifluoromethanesulfonyl),
THICA — trihaloisocyanuric acids,
Ts — tosyl.
Funding
This review was written with the financial support from the Russian Foundation for Basic Research (Project No. 20-03-00700А).
References
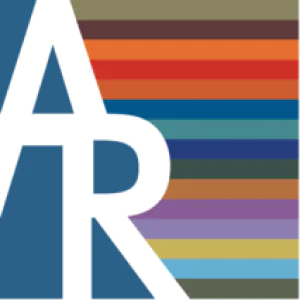
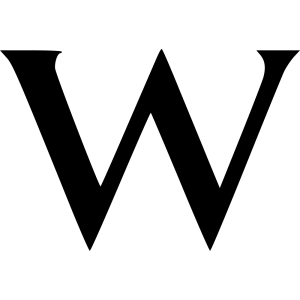
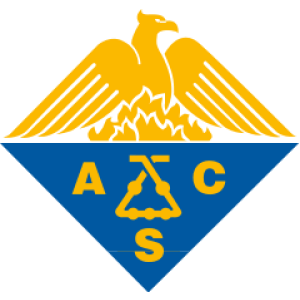
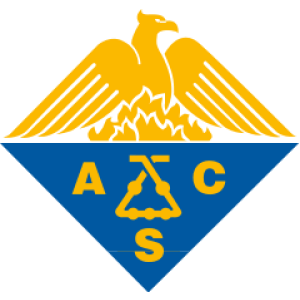
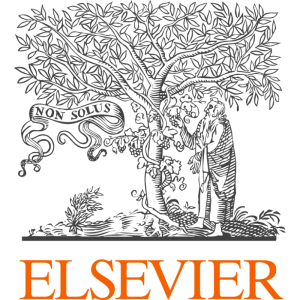
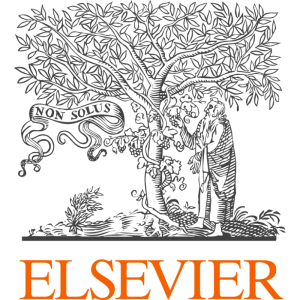
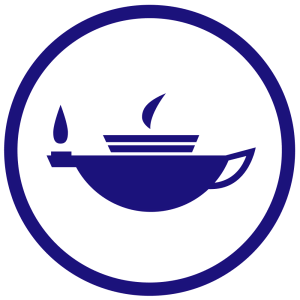
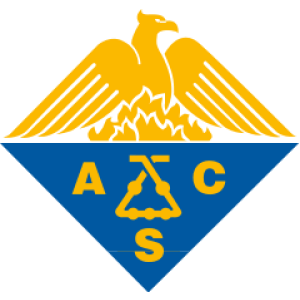
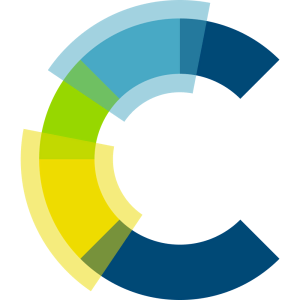
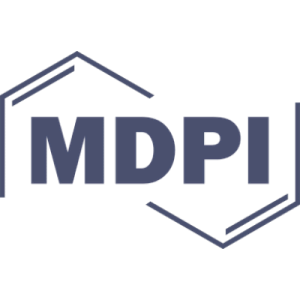
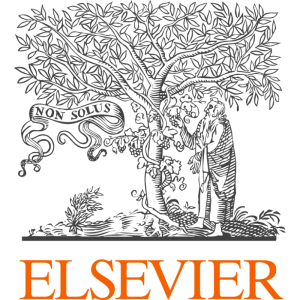
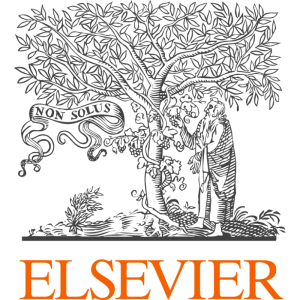
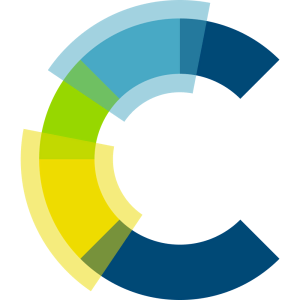
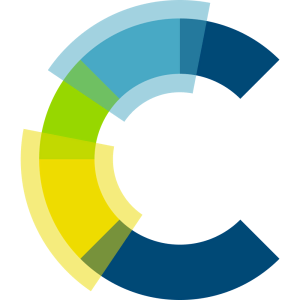
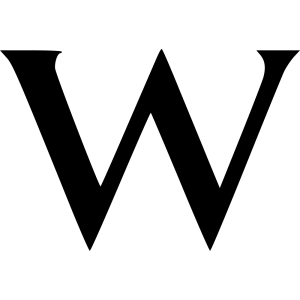
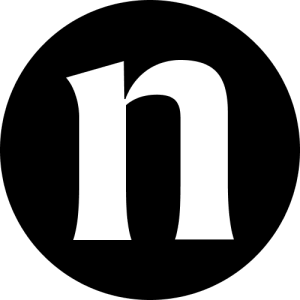
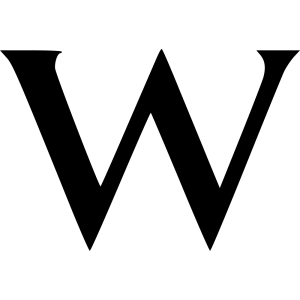
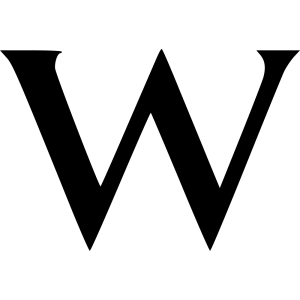
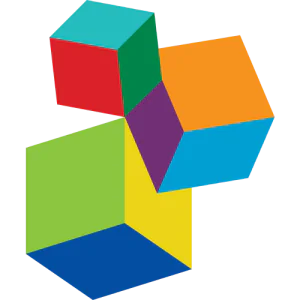
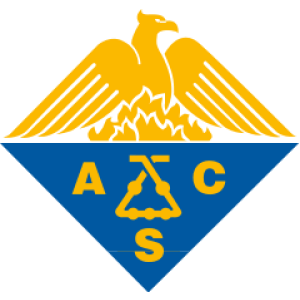
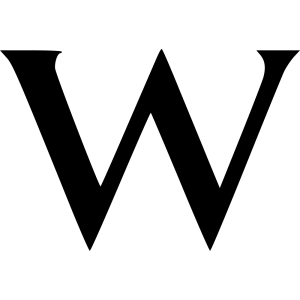
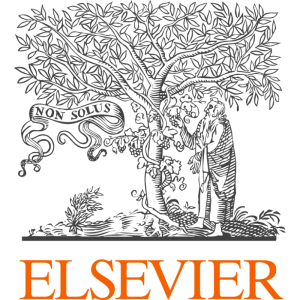
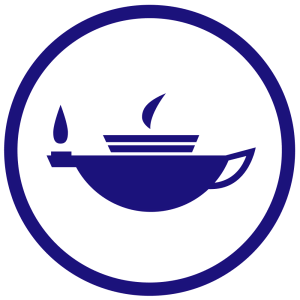
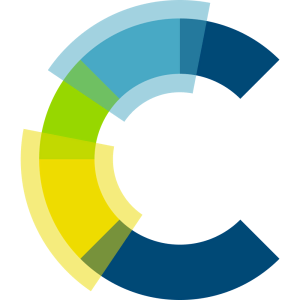
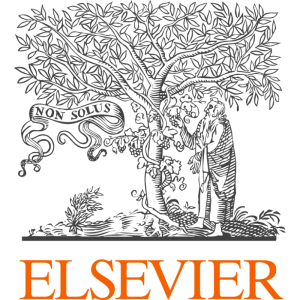
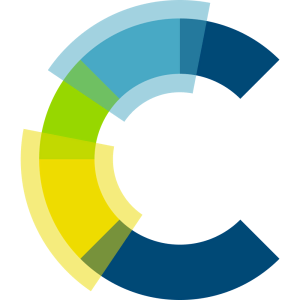
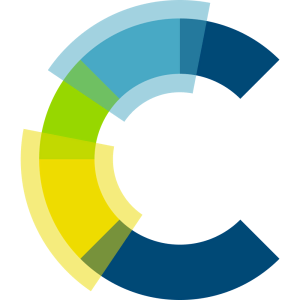
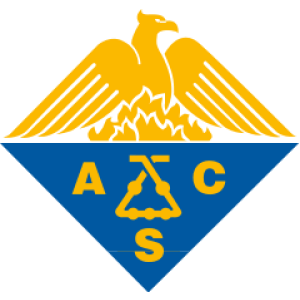
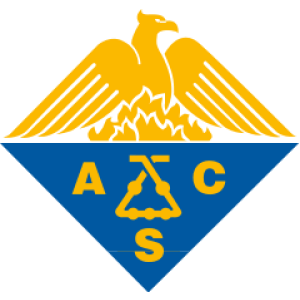
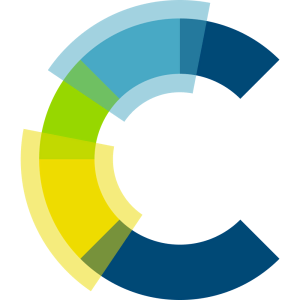
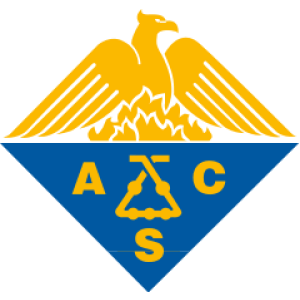
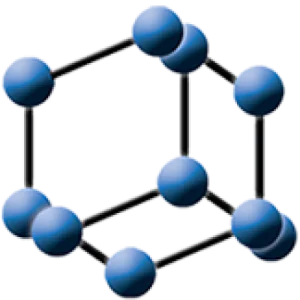
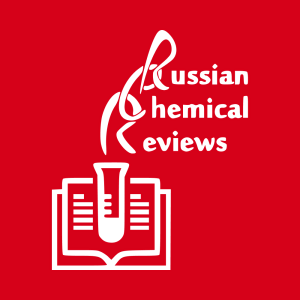
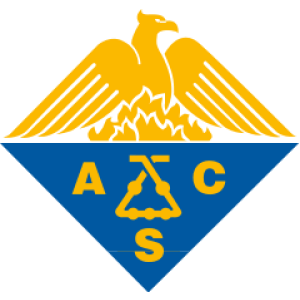
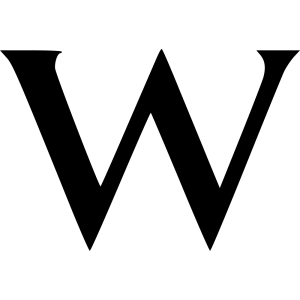
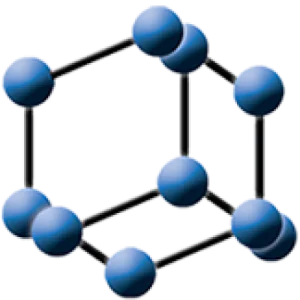
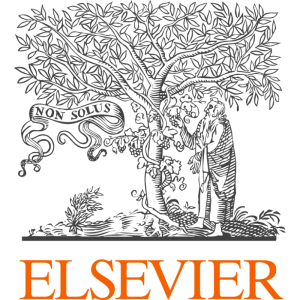
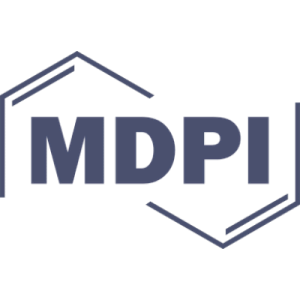
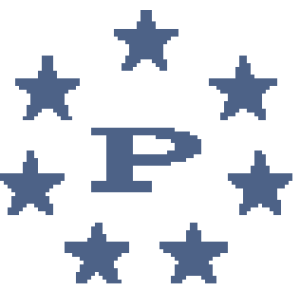
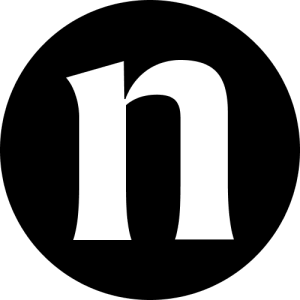
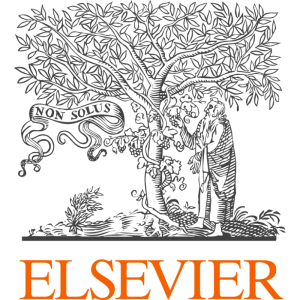
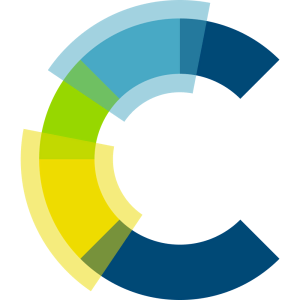
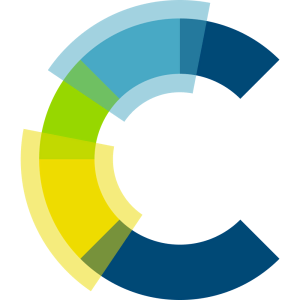
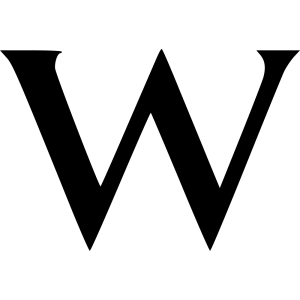
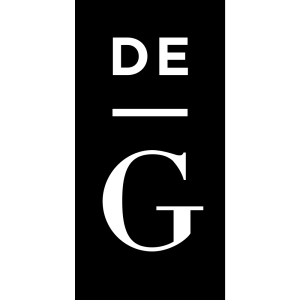
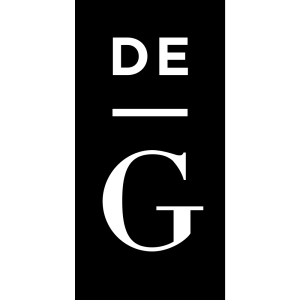
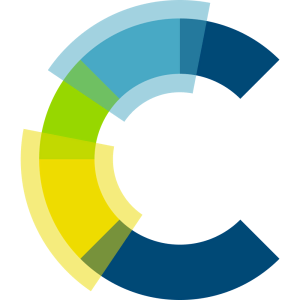
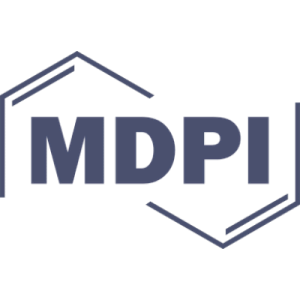
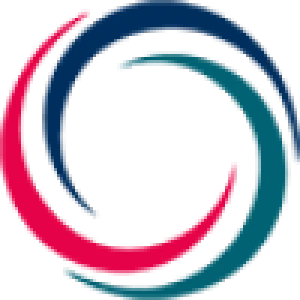
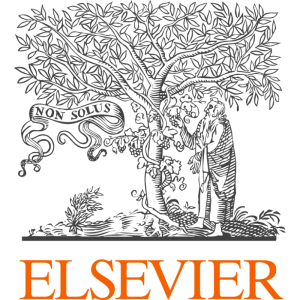
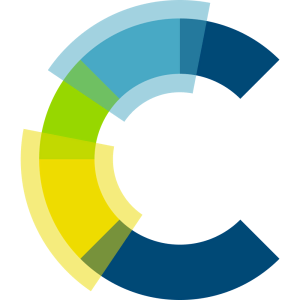
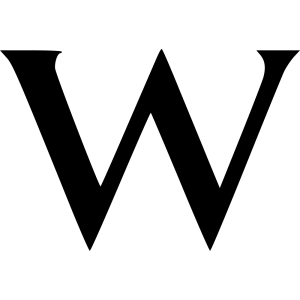
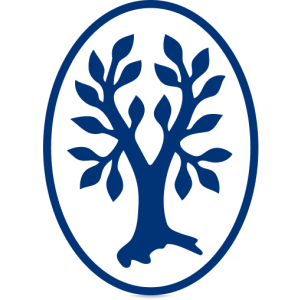
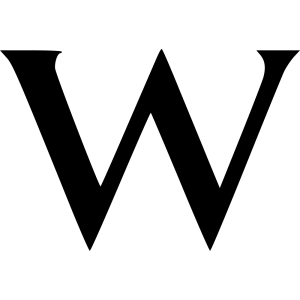
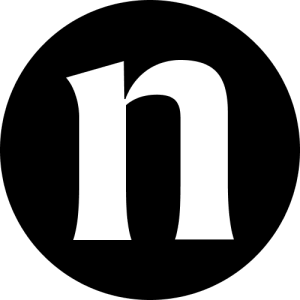
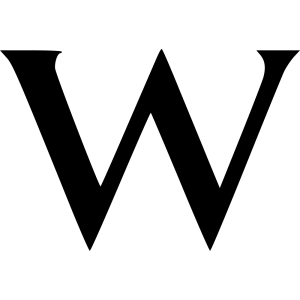
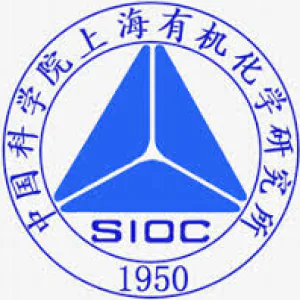
![An efficient approach for the synthesis of 5-hydroxy-chromeno[2,3-b]pyridines under catalyst and solvent free conditions](/storage/images/resized/leiAYcRDGTSl5B1eCnwpSGqmDEUEfDPPoYisFGhT_small_thumb.webp)
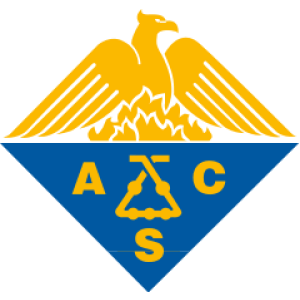
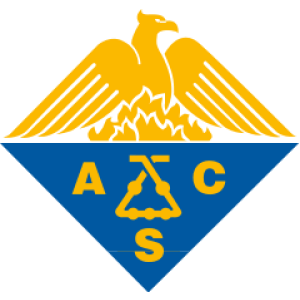
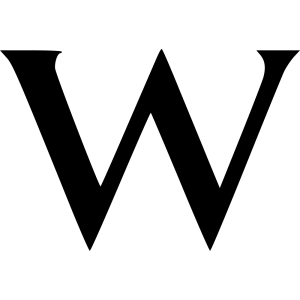
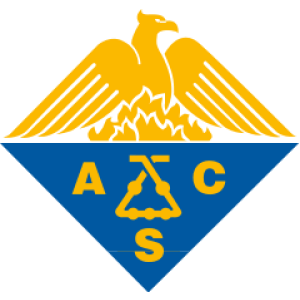
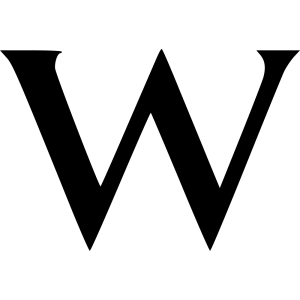
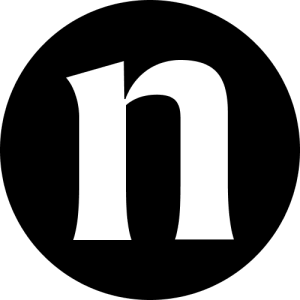
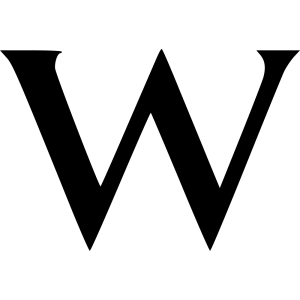
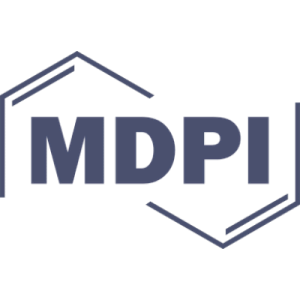
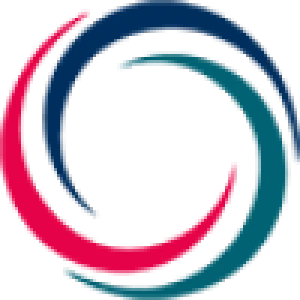
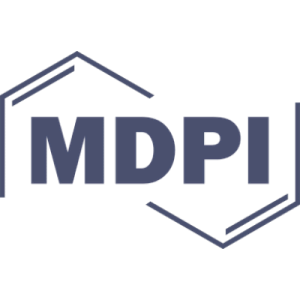
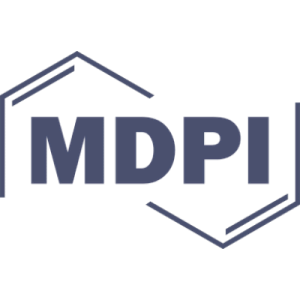
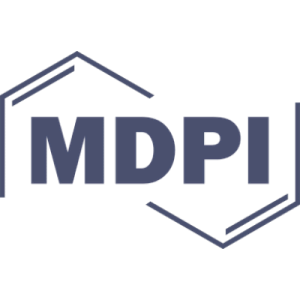
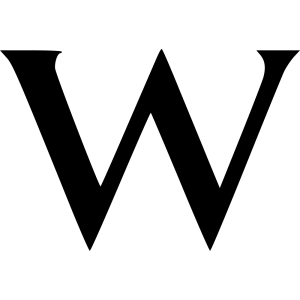
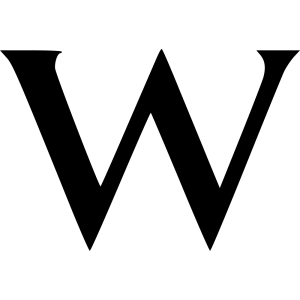
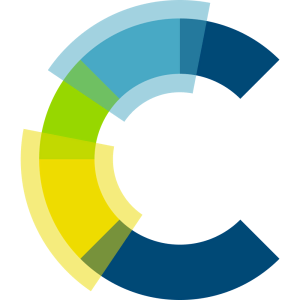
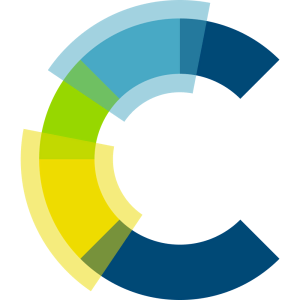
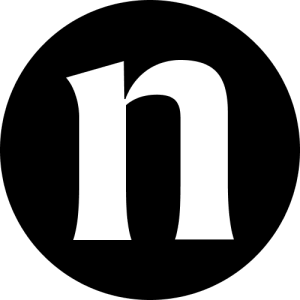
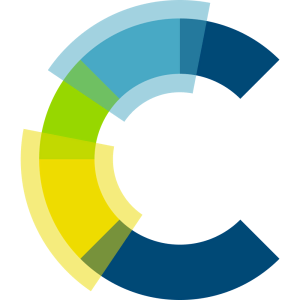
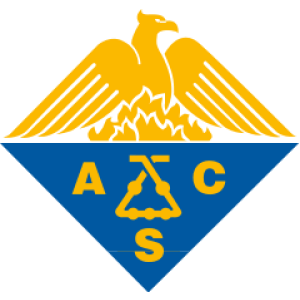
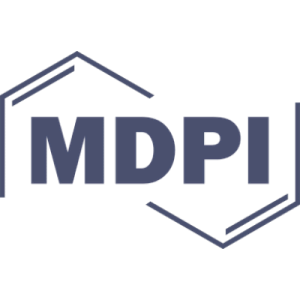
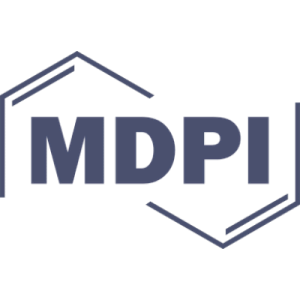
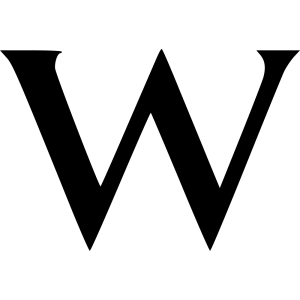
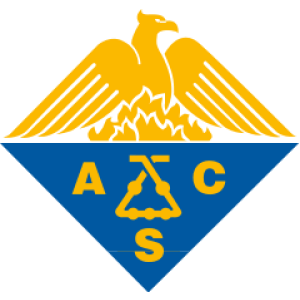
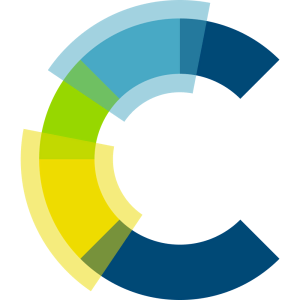
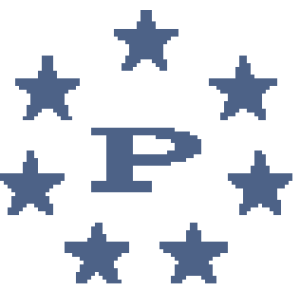
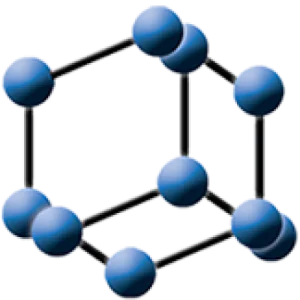
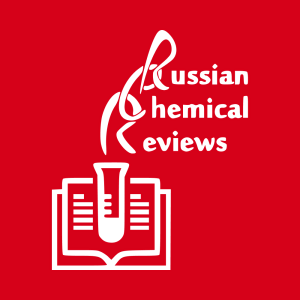
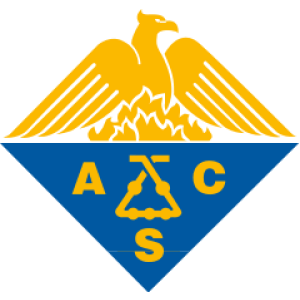
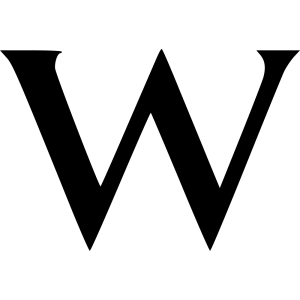
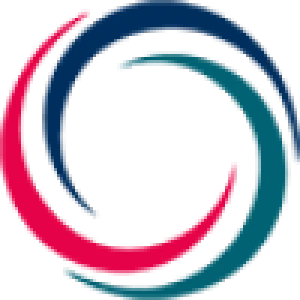
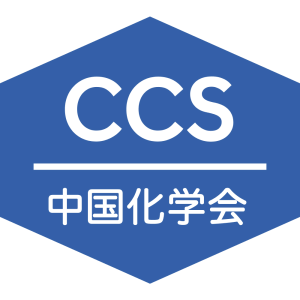
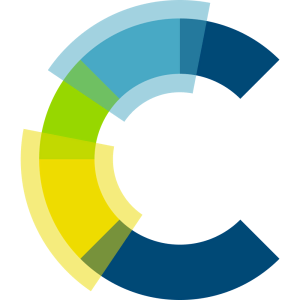
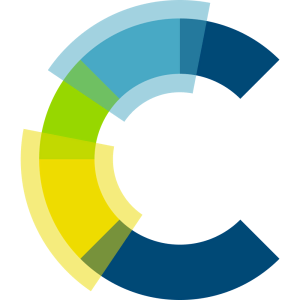
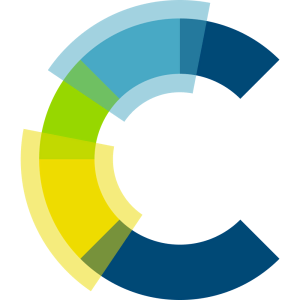
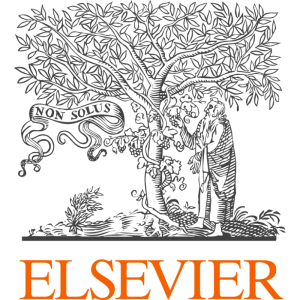
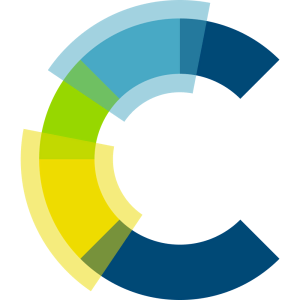
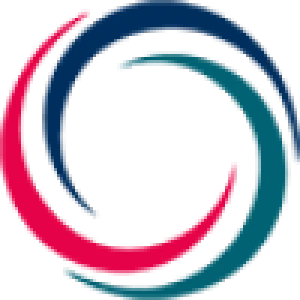
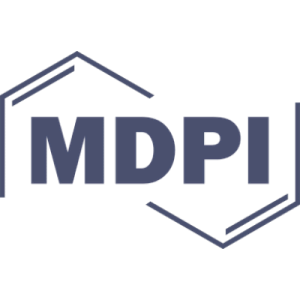
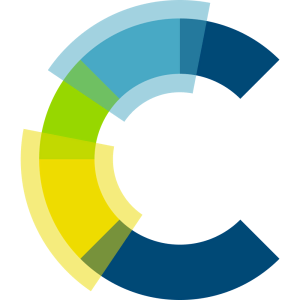
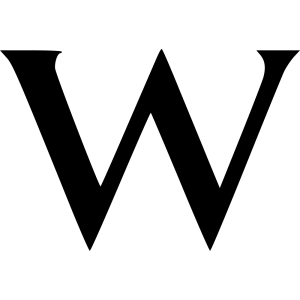
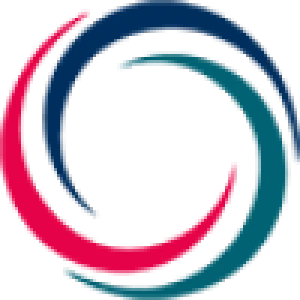
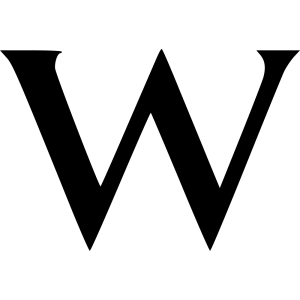
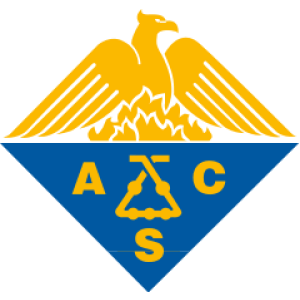
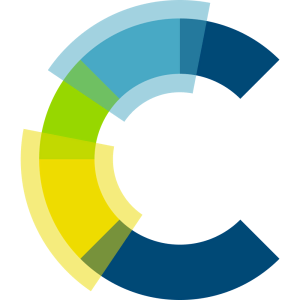
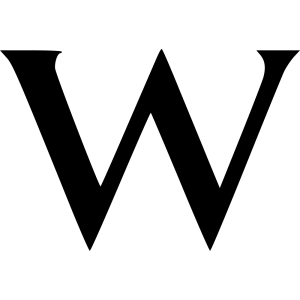
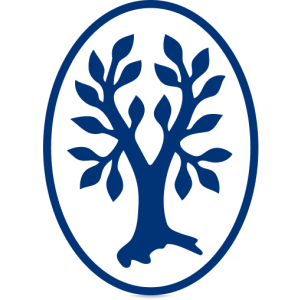
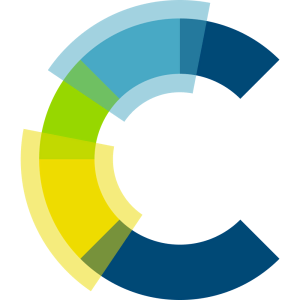
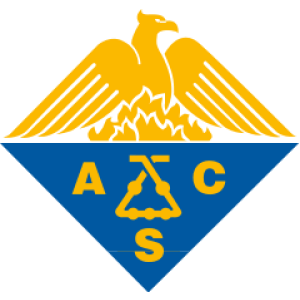
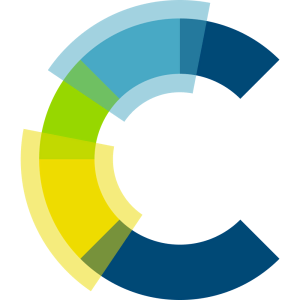
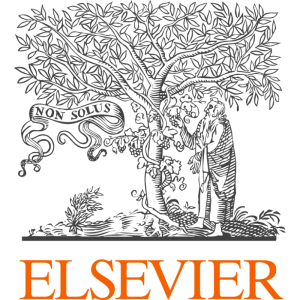
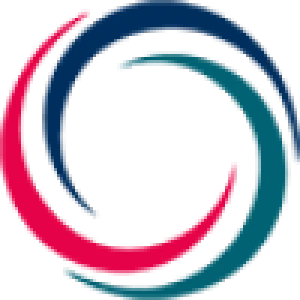
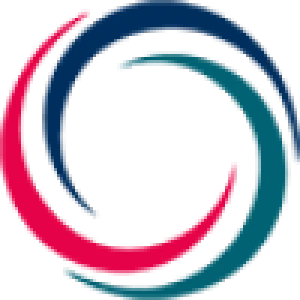
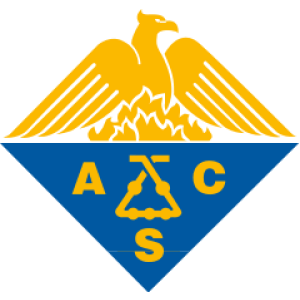
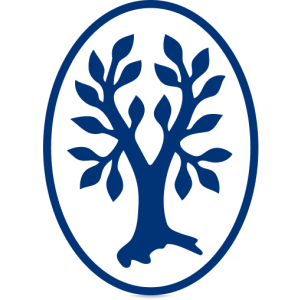
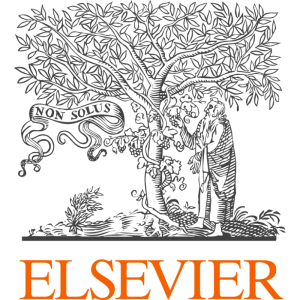
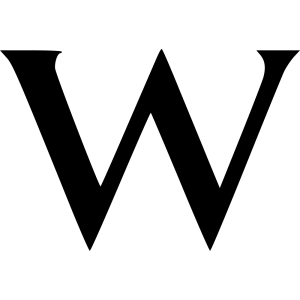
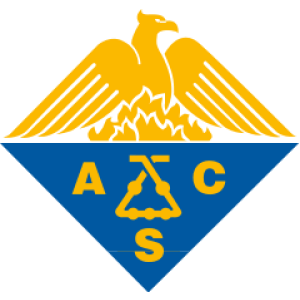
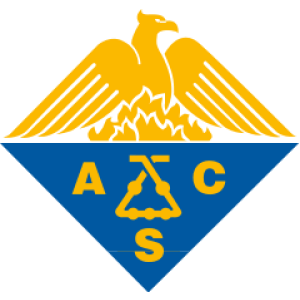
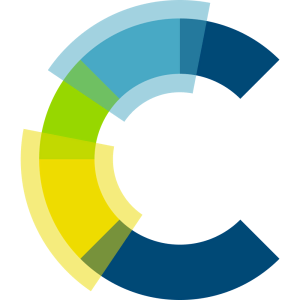
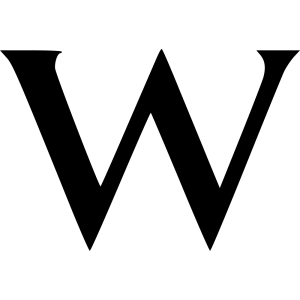
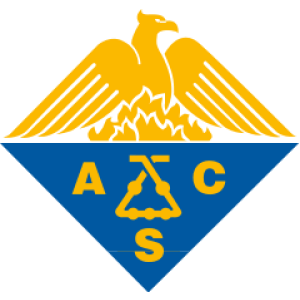
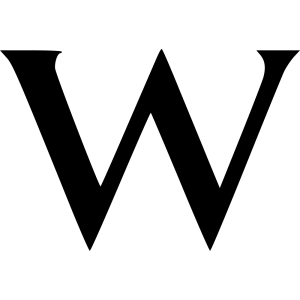
![[Cp*RhCl2]2: mechanosynthesis and applications in C-H bond functionalisations under ball-milling conditions.](/storage/images/resized/leiAYcRDGTSl5B1eCnwpSGqmDEUEfDPPoYisFGhT_small_thumb.webp)
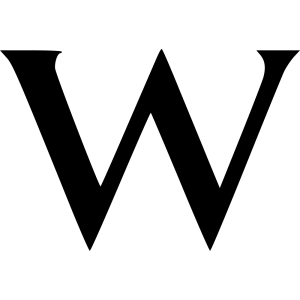
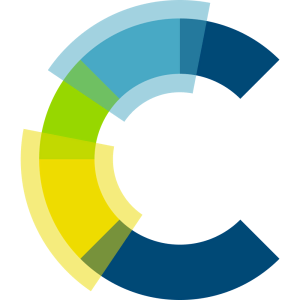
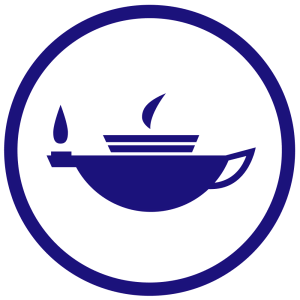
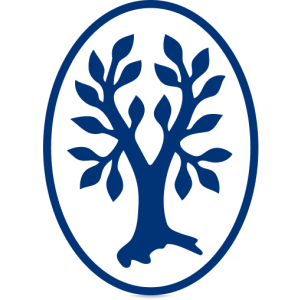
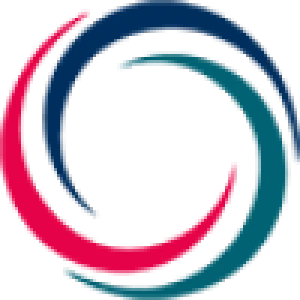
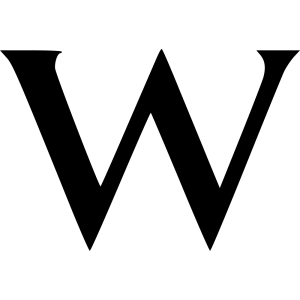
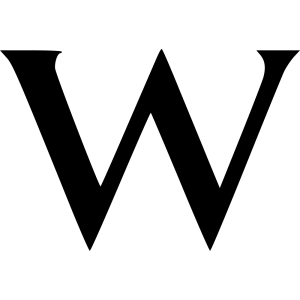
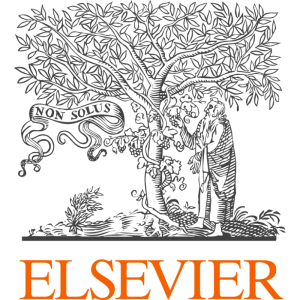
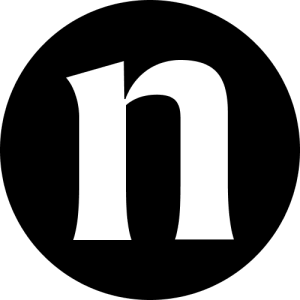
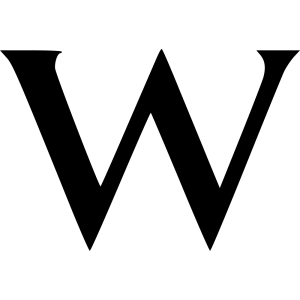
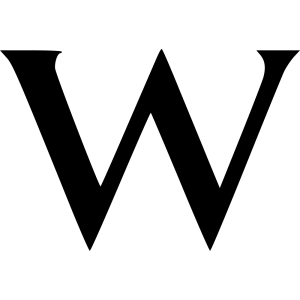
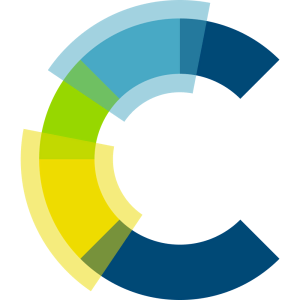
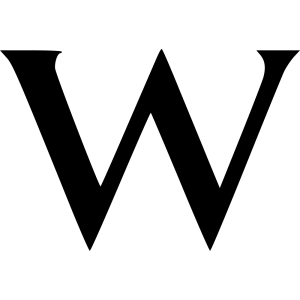
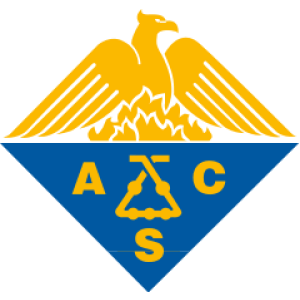
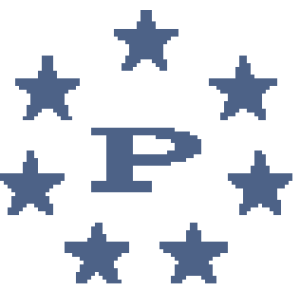
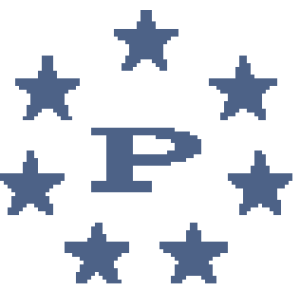
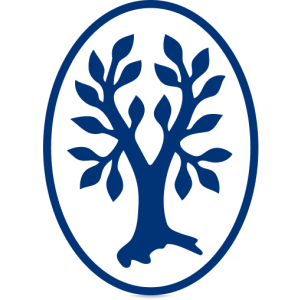
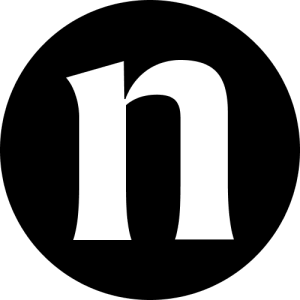
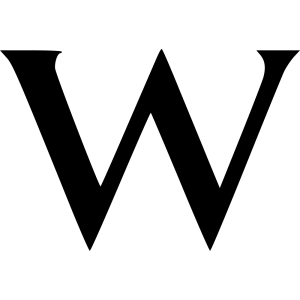
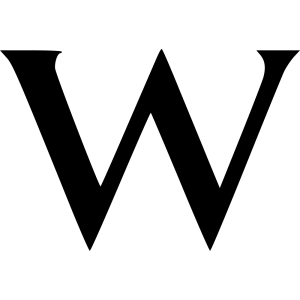