Keywords
Abstract
The review presents a summary and systematic analysis of the literature data on the antibacterial and antifungal activity of palladium complexes with organic ligands published over the past three years. The structures of different types of stable complexes are discussed. Taking into account the great structural diversity of the compounds under consideration, the classification of the complexes is performed in terms of the nature of donor centres of the initial ligands. A group of palladium phthalocyanine complexes for photodynamic therapy is considered separately. The final section is devoted to palladium complexes with terpene ligands, which are of scientific interest to the authors of this review.
The bibliography includes 97 references.
1. Introduction
Almost all publications on the evaluation of antimicrobial activity of metal complexes begin with the discussion of a pressing problem of the rapidly growing resistance of abundant pathogenic strains to available clinical antibiotics and antifungal drugs. According to the World Health Organization,† antibiotic resistance is one of the top ten global public health threats to humanity in the 21st century.
The international scientific team with the participation of Australian researchers has made significant progress in addressing this problem[1]. Within the framework of the not-forprofit initiative Community for Open Antimicrobial Drug Discovery (CO-ADD), a large number of metal-containing compounds were screened for antimicrobial activity. Out of 906 compounds, 88 were found to show activity against at least one of the tested strains, including fungi, while displaying no cytotoxicity against mammalian cell lines. An analysis of the results of the publication[1] demonstrated that palladium complexes are in the top three of 16 tested groups of compounds in terms of activity. For example, palladium compounds account for 14% of active and nontoxic agents, being second only to platinum complexes (18%). Unfortunately, palladium complexes have not been adequately addressed in the study[1]. In the review[2], the prospects of metal complexes as antimicrobial agents are also considered, but no data on palladium compounds are reported. The lack of information stimulated us to analyze the studies aimed at evaluating the antimicrobial activity of palladium complexes, which were published in 2021–2023, summarize and systematize these results. We limited this review to the past three years because there is a number of previous review papers[3-8], in which the results of the evaluation of metal complexes (including palladium compounds) as antibacterial and antifungal agents are summarized. The knowledge of the types of active compounds is important for the design of new antibiotics with high antimicrobial potential.
The general strategic issues of the design of effective antibiotics require a knowledge of the target of the attack, the molecular target, and a possible mechanism of action[5, 9]. Bacteria are prokaryotic cell organisms, which do not contain certain cell organelles compared to eukaryotic cells. Bacteria do not have a nucleus, and their genetic material is found in the cytoplasm in the form of nucleotide. Besides, these organisms are classified as either Gram-positive or Gram-negative, which is attributed to the structure of their cytoplasmic membranes. Gram-positive bacteria have a cell wall composed of a relatively thick peptidoglycan layer. Gram-negative bacteria have a difficult-toaccess plasma membrane composed of lipopolysaccharides and use a porin (efflux) pump to export the required compounds into the cell and remove any unwanted waste from the cell. There are also other organelles found in both Gram-positive and Gramnegative bacteria, for example, a polysaccharide membrane called a capsule around their outer membrane. This capsule prevents the phagocytosis of bacteria by other organisms or human phagocytic cells. Bacterial cells have a cytoplasm, in which cellular processes take place. The cytoplasm contains all important cell organelles and macromolecules, such as ribosomes, plasmids and chromosomes. Bacterial cells also contain a cytoplasmic membrane consisting of proteins and phospholipids. This membrane is important for bacteria, controling the flow of molecules into and out of the cells. Some bacteria have flagella, which are whip-like structures, responsible for bacterial locomotion. Since bacteria have a single chromosome, their double-stranded DNA is located in the membrane-free region known as the nucleoid. Most of bacteria contain pili, which are whip-like appendages on the outer membrane of bacterial cells that help bacteria attach to surfaces. Finally, all bacteria have ribosomes that translate their genetic code.
Therefore, bacteria have multiple targets and each class of antibacterial agents is characterized by a specific mode of action against bacterial cells. Certain agents cause the mechanical disruption of the structures of bacterial cells, e.g., of the cell membrane. Other agents act on bacteria through inhibition of the biosynthesis of particular macromolecules that are produced during bacterial cell division, growth and differentiation. Bacteria resist antibiotics through two main mechanisms: by decreasing the concentration of the antibiotic before it reaches the target or by changing the structure of bacterial organelles, that are antibiotic targets, in order to prevent the cell death.
In the review[9], Boros et al. emphasized that the understanding of the mechanism, by which metal compounds exert their biological effects, and the knowledge of the key parameters required for enhancing these properties will allow the more rational design of new compounds. For example, palladium complexes can justifiably be compared with the known platinum-based clinical anticancer drugs, such as cisplatin, carboplatin and oxaliplatin. One of the key characteristics of many metal complexes is the active chemical ligand exchange responsible for the mechanism of action of the well-known metal-based agents used in the clinical practice, namely PtII complexes. In fact, the metal ion is covalently bound to important biomolecules, e.g., DNA, proteins, enzymes, etc., inhibiting their function and leading to different types of cell death (e.g., through the apoptosis and necrosis). After the intravenous infusion of cisplatin, the complex remains to a large extent unchanged due to a high concentration of chloride ions in the blood. After entering the cell, the complex undergoes aquation, i.e., one or two ligands (chloride ions) are replaced by water molecules (because the chloride concentration in the cell is significantly lower than that in the blood). The resulting PtII particles are activated and then are bound to nuclear DNA, predominantly to the N(7) atom of guanine, to form mainly intrachain cross-links. These cross-links block the replication and cell division, interfering with RNA processing. Based on this mechanism, cisplatin and related DNA-binding agents are referred to as alkylating agents; in organic medicinal chemistry, these agents are termed as covalent inhibitors.
With a few exceptions, the studies on the comparative evaluation of biological activity of metal complexes and the corresponding initial ligands demonstrated that the complexes are significantly more active than the ligands. This is evidence of an important role of the metal ion in the mechanism of antimicrobial or anticancer activity of metal-containing agents. Numerous examples can be found below. Most of the authors explained this fact as follows. The complexation leads to an increase in the lipophilicity of transition metal ions due to the delocalization of π or p electrons of the ligands in the metal coordination sphere. This, in turn, improves the diffusion of the complex molecule through the cell membrane. Although the chelation plays an essential role in the antimicrobial behaviour of the complexes, other factors can also enhance and control this activity of metal complexes compared to the free ligands. These factors include the size and geometry of the complex, its dipole moment, the solubility, the nature of the donor centre, the redox potential of the metal ion, the bond length between the ligand and the metal, steric and pharmacokinetic parameters and the concentration of the compound. Besides, the changes in the behaviour of metal complexes towards different microbes can depend on the difference in the cell wall permeability of microorganisms.
This review presents examples of different types of palladium complexes with known antibacterial and antifungal activity. Selected results of relevant studies are given in the tables, the most significant activity values being highlighted in bold. These data demonstrate that palladium complexes are promising antimicrobial agents
2. Palladium complexes with N- and O-donor ligands
Chemical modification of structures of the known drugs is a promising strategy for discovering more effective compounds in a particular pharmaceutical group, called ‘the next in the class’. For example, three palladium complexes (Pd1–Pd3, Table 1) containing ofloxacin (Figure 1.) and the amino acids glycine or alanine as the ligands were evaluated as antimicrobial materials[10].
Ofloxacin is a broad-spectrum second-generation fluoroquinolone antibiotic against anaerobic and aerobic microorganisms and a specific inhibitor of bacterial DNA gyrase. The antibacterial activity of complexes Pd1–Pd3 was evaluated against Klebsiella pneumoniae and Escherichia coli strains. Ofloxacin was used as the reference drug (positive control), which proved to be inactive in these experiments.‡ The coordination of ligand L1 to palladium(ii) gives rise to complexes Pd1–Pd3 exhibiting bactericidal activity. It is worth noting that the activity of homoleptic complex Pd1 is somewhat higher than that of mixed-ligand complexes Pd2 and Pd3. For comparison, the authors cited the data for ofloxacin-based RuIII complexes obtained previously[11], which showed that it is preferable to use the corresponding palladium compounds.
Ethylene- and propylenediamine derivatives were extensively studied as N,N-donor chelating ligands by Serbian researchers[12-14]. Chiral ligands containing amino acid moieties and their complexes with palladium are also of obvious interest. A wide screening of the antimicrobial activity of complexes Pd4–Pd7 and the corresponding ligands containing a tryptophan moiety was performed (Table 2)[12]. The authors used 19 microorganisms: 15 bacterial strains, including three probiotics, five standard strains and seven isolates, as well as four types of yeast. The antibacterial activity of the tested ligands and complexes was quite selective. The distinguishing feaeture of this group of compounds is that the activity of the complexes is very similar to the activity of the corresponding ligands, except for compounds L6 and L7. The latter exert a stronger effect on Gram-negative bacteria and yeast compared to their complexes. Bacterial E. coli cells showed the highest sensitivity to all the tested complexes and ligands. The minimum inhibitory concentration (MIC) of complex Pd6 is 7.81 μg mL–1, which is significantly higher than the activity of tetracycline (15.63 μg mL–1). It is worth noting that there is no clear dependence of the antibacterial activity of the tested compounds on the length of the hydrophobic alkyl group (R = Et, Prn, Bun, n-C5H11).
Propylenediamine ligands containing O-alkylated valine moieties were used to synthesize chiral complexes Pd8–Pd10[13]. The antimicrobial activity of these ligands and complexes was evaluated against 12 microorganisms. Generally, the tested compounds exhibit selective and moderate activity. The difference in the antimicrobial activity of the ligands and the corresponding complexes Pd8–Pd10 is insignificant. The MIC values vary from 31.25 to >1000 μg mL–1, but these compounds exhibit low antibacterial activity compared to the positive control (MIC of tetracycline varies from 0.11 до 62.5 μg mL–1, which is indicative of the selectivity of action of the agent on different strains).
Bis(pyridyl)allene ligands can be assigned to ‘exotic’ scaffolds for the preparation of metal complexes[15]. These ligands provide an approach to compounds with axial chirality. Complexes Pd11 and Pd12 were synthesized based on racemic ligands. Within the framework of the CO-ADD project, the complexes and the corresponding ligands were tested for the antimicrobial activity against the following panel of microorganisms: S. aureus ATCC 43300, P. aeruginosa ATCC 27853, E. coli ATCC 25922, K. pneumoniae ATCC 700603, Actinetobacter baumannii ATCC 19606, Cryptococcus neoformans and Candida albicans. The primary screening of the antimicrobial activity showed that, when taken at a single concentration of 32 μg mL–1, complexes Pd11 and Pd12 are quite active (the inhibition of the growth of microorganisms was higher than 80%). The dose-dependent experiment demostrated that complex Pd12 is a lead compound (MIC against S. aureus, C. albicans and C. neoformans<0.25 μg mL–1). Complexes Pd11 and Pd12 are characterized by high toxicity towards the human embryonic kidney cell line HEK293. The CC50 value § of these complexes against the cell line НЕК-293 was ~1 μg mL–1.
Two tridentate pyridine-based ligands, benzimidazole and tetrapyridine, were used to synthesize ionic palladium complexes (Pd13, Pd14) and platinum complexes (Pt13, Pt14)[16]. The antimicrobial screening of the metal complexes was performed by CO-ADD according to the standard protocol. Although these compounds exhibited low antibacterial activity, their antifungal activity deserves attention. Palladium complex Pd13 with the benzimidazole ligand showed the best inhibitory activity against the fungi C. neoformans and C. albicans (MIC was 1 and 0.5 μg mL–1, respectively), while MIC of the ligand was >32 μg mL–1. Triazole derivatives of pyridine containing perfluoroalkyl substituents and their complexes Pd15 and Pd16 were synthesized in order to evaluate their bactericidal activity[17]. Both complexes exhibited high activity against the Gramnegative bacteria E. coli. Thus, the zone of inhibition was about 80–90 mm (in the presence of 5 μmol L–1 (μm) compound), which is larger than that of vancomycin (60 mm) and is comparable with that of ampicillin (100 mm).
Imidazo[1,2-a]pyridine was successfully used as a precursor for the preparation of broad-spectrum drugs[18]. The bactericidal activity of three palladium(ii) complexes of the composition PdL2Cl2 (Pd17), [PdL4]Cl2 (Pd18) and [PdL4]Cl2(4H2O)L (Pd19), where L is imidazo[1,2-a]pyridine, was evaluated against the microorganisms S. aureus, B. subtilis, B. spizizenii, E. coli and P. aeruginosa. It was found that complex Pd19 exhibited moderate bactericidal activity against S. aureus; the MIC value and the minimum bactericidal concentration (MBC) were 18.75 μg mL–1 (22 μm) and 75.00 μg mL–1 (89 μm), respectively. Two other compounds (Pd17 and Pd18) proved to be inactive against the whole range of the tested pathogenic strains. Although the authors gave no explanation for these observations, we agree with their optimistic conclusion that this ‘finding may encourage other researchers to join in designing newer palladiums as antibiotics’.
Aryl-substituted biguanidine ligands can be used to synthesize bischelate ionic metal complexes[19]. Such complexes (Ni20, Pd20 and Pt20) were evaluated as antimicrobial agents against the following strains: E. coli ATCC 25922, P. aeruginosa ATCC 27853, S. aureus ATCC 29213, B. subtilis ATCC 6633, Enterococcus faecalis ATCC 29212 and C. albicans 22 (Table 3). The metal complexes exhibited significantly higher activity compared to ligand L20 used as hydrochloride. The palladium complex proved to be the best inhibitor of the growth of the bacteria S. aureus, B. subtilis, E. faecalis and C. albicans.
Schiff bases are the most popular ligands used for the synthesis of metal complexes. Iminocoumarin ligands and their complexes Pd21–Pd24 were synthesized and tested as antimicrobial agents[20]. The antibacterial activity was evaluated against the strains E. сoli ATCC 25922, K. pneumoniae ATCC 13883, S. aureus ATCC 25923 and E. faecalis ATCC 19433. The ligands and complexes were found to exhibit weak antibacterial activity against the Gram-positive bacteria. For complexes Pd21–Pd24, the diameter of the growth inhibition zone of the Gram-positive bacteria was in the range of 10–14 mm. The Gram-negative bacteria proved to be insensitive to the tested compounds. This fact was attributed to the difficulty of penetration of the agent through the lipid layer of these bacteria. A significant improvement of the antifungal activity against the strains C. albicans ATCC 24433, C. tropicalis ATCC 10233 and Aspergillus niger ATCC 135550 was observed when using complexes Pd21–Pd24 instead of the corresponding ligands. For example, the growth inhibition zone of the fungi C. albicans in the presence of compounds Pd22 and Pd24 containing more polar groups are in the range of 20–40 mm; of the corresponding ligands, ~ 10 mm.
Complexes Pd25, Co25, Ni25, Cu25 and Zn25 with the azomethine ligand contain a polar carboxyl group responsible for their hydrophilicity (Table 4)[21]. Different types of biological activity, including antimicrobial activity, of these compounds were studied. Complex Pd25 was found to have a broad spectrum of antibacterial activity against Proteus vulgaris, K. pneumoniae, P. aeruginosa, S. aureus and B. subtilis strains. In all experiments, the coordination of the ligand to palladium led to the enhancement of antimicrobial activity. In terms of activity, palladium complex Pd25 is second only to the related copper complex (Cu25).
Compounds M26, where М = Ni, Cu, Zn, Pd, Cd or Hg[22], are examples of metal complexes containing a N,N-donor ligand with a coordination mode similar to that described above. The distinguishing feature of these complexes is the presence of a phosphoric acid moiety. The antibacterial activity of these compounds was evaluated against the microorganisms S. aureus and E. coli. Only qualitative results of the evaluation were reported, which are indicative of a low inhibitory activity of complex Pd26; the best results were observed for its analogue Ni26.
Hydrazones containing the diazine core were synthesized and used to prepare complexes Pd27–Pd31 containing different halogen substituents on the benzylidene moiety (Table 5)[23]. The antimicrobial screening of this series of compounds was carried out against the bacterial strains of S. aureus, B. subtilis, E. coli and P. aeruginosa, the fungi C. albicans and the yeast Saccharomyces cerevisiae. In all tests, the ligands were significantly less active than the corresponding palladium complexes. The bactericidal activity of complexes Pd27–Pd31 against the S. aureus, B. subtilis and P. aeruginosa strains was comparable to that of ciprofloxacin used as the reference drug. The Gram-negative bacteria E. coli were less sensitive to these agents. In this series, the clear structure–property relationship was not revealed. Complexes Pd27–Pd31 can be considered as effective antimicrobial agents.
Metal complexes with azomethine ligands were extensively studied by international teams of United Arab Emirates and Egyptian researchers[24-29]. Complexes Cu32, Pd32 and Ag32 with 2-[(4-chlorobenzylidene)amino]phenol (L32) were synthesized (Table 6)[24]. The antibacterial activity of these compounds was evaluated against three bacterial strains of E. coli, B. subtilis and S. aureus; the antifungal activity, against C. albicans, A. flavus and Trichophyton rubrum. In this series of three metal complexes, Pd32 did not exhibit satisfactory activity.
The best results were observed for compound Ag32. In the study[24], a significant effect of the coordination to metal was demonstrated once again. Thus, free ligand L32 was almost inactive.
A similar dependence was observed for complexes М33, where М = Cu, Pd or Ag, based on the tetradentate salen-type ligand (Table 7)[25].
However, in another series of three metal complexes (Pd34, VO34 and Ag34)[26], which were synthesized based on 1-(3-pyridyliminomethyl)-2-naphthol (HL34), the palladium complexes were found to be the lead compounds (Table 8). These compounds were tested in vitro against three bacteria (E. coli, Serratia marcescens, Micrococcus luteus) and three fungi (Geotrichum candidum, A. flavus, Fusarium oxysporum). The antimicrobial activity of azomethine ligand HL34 was found to be significantly lower than the activity of its metal complexes. Complex Pd34 proved to be the most effective. It showed the lowest MIC values against the tested bacteria and fungi compared to the other compounds.
Complex Pd35 with a related ligand, which was synthesized in the study[27], has an unusual structure consisting of six- and four-membered palladacycles. The screening demonstrated that complex Pd35 exhibited the strongest inhibitory activity against the growth of the bacteria S. marcescens, E. coli and M. luteus (MIC was 3.25, 3.75 and 3.00 μM, respectively) compared to the related zinc(ii), chromium(iii) and vanadyl(ii) complexes. Structure Pd36, proposed for the PdII complex with quinoline imine[28], seems to be more reliable than the above formula Pd35. In the study[28], a comparative analysis of the antimicrobial and antifungal activity of compound Pd36 and related ZnII, CrIII and VOII complexes was performed, and the palladium derivative was found to have advantages because it inhibited the growth of the bacteria S. marcescens, E. coli and M. luteus (MIC was 2.25, 2.75 and 1.50 μM, respectively). High biological activity of PdII complexes was confirmed by molecular docking, assuming that the square-planar geometry of the palladium centre promotes a more efficient interaction with the active sites of biomolecules.
Transition metal complexes were synthesized based on the azomethine ferrocenyl ligand (Table 9)[29]. The screening of biological activity of complexes M37, where М = Mn, Pd, Zn or VO, and uncoordinated ligand HL37 was carried out against pathogenic bacterial (S. marcescens, E. coli and M. luteus) and fungal (A. flavus, G. candidum and F. oxysporum) strains. As expected, free ligand HL37 showed lower antimicrobial activity than the corresponding metal complexes. The results of this study demonstrated that complex Pd37 possessed the best antibacterial and antifungal activity compared to the other complexes, which is comparable with the activity of ofloxacin and fluconazole used as the reference drugs.
Fluorine-containing salen-type imine was used as the ligand for the synthesis of chelate complexes Cu38, Ni38 and Pd38 (Table 10)[30]. In this series of three compounds, complex Pd38 exhibited higher antimicrobial activity against both pathogenic bacterial strains (E. coli, S. aureus, P. aeruginosa) and fungal microorganisms (A. niger, A. flavus, C. albicans). This difference of complex Pd38 was attributed to its high antioxidant activity established in this study as well. Amali et al.[30] suggested that the resulting reactive oxygen species (ROS) and metal ions attack the negatively charged cell wall of bacteria and lead to its disruption, which causes death of microbes.
Halogen-substituted quinolinols, 5-chloro-8-hydroxy-7- iodoquinoline (HL39), 5,7-dichloro-8-hydroxyquinoline (HL40) and 5,7-dibromo-8-hydroxyquinoline (HL41), which are used as ligands for the coordination to metals, are known as antiviral, antibacterial, antifungal and antiprotozoal drugs[31]. In order to determine the metal complex state of these ligands, the authors synthesized a series of ionic palladium complexes Pd39–Pd41 of the general formula Cat[PdCl2(L)] (Cat = K or Cs) and evaluated their antimicrobial activity against 19 strains of pathogenic microorganisms, including 15 bacteria and 4 fungi (Table 11, selected data are given)[31]. As opposed to most of the above results, the activity of the initial ligands appeared to be comparable with the activity of the corresponding metal complexes. The test using P. aeruginosa strains was the only example, in which the complexes were more active than the ligands. The yeast strains were the most sensitive to the presence of these compounds. The Gram-positive bacteria showed higher sensitivity to these compounds compared to the Gram-negative bacteria. No clear dependence of the antibacterial activity on the nature of the substituents and the nature of the cation was revealed.
Mononuclear complexes Pd42–Pd44 containing nicotinamide (L42), picolinic acid (L43) and isonicotinic acid (L44) as the ligands and mixed-ligand analogues Pd45a–c containing the caffeine moiety (L45) were screened for antibacterial activity[32]. The experiments demonstrated that all the tested compounds exhibited no antibacterial activity against four bacterial strains (K. pnuemoniae, E. coli, S. aureus and S. epidermidis). Despite the negative results, this study deserves mention because any data are important for the comparative structural analysis.
The introduction of a pharmacophoric moiety into the target structure is a promising approach to the design of effective drugs, which was used in the study[33]. The aim of this study was to synthesize and characterize three new palladium complexes with Schiff bases (Pd46–Pd48), which were prepared from pyrrole-2-carboxaldehyde and L-methionine, L-histidine or L-tryptophan, and test their antibacterial activity (Table 12). The antibacterial activity of the compounds was evaluated against Gram-positive (S. aureus ATCC 25923, MRSA ATCC 33591, S. epidermidis ATCC 12228 and Streptococcus pyogenes ATCC 19615) and Gram-negative bacteria (P. aeruginosa ATCC 27853 and K. pneumoniae ATCC 13883). It was found that the ligands inhibited the growth of none of the tested bacterial strains. The MIC values of all ligands were lower than 2.5 mg mL–1. The lowest MIC value (0.078 mg mL–1) was observed for complex Pd48 against the microorganisms S. epidermidis, S. aureus and the methicillin-resistant Staphylococcus aureus strain (MRSA). A similar MIC value was observed for compound Pd47 against S. epidermidis. In all other cases, the MIC values were higher than 0.078 mg mL–1. The highest MIC value (0.625 mg mL–1) was found for complex Pd46 against the S. pyogenes, S. aureus and MRSA strains. An analysis confirmed that P. aeruginosa is resistant in the presence of all the tested complexes Pd46–Pd48. The higher antibacterial activity of compounds Pd47 and Pd48 was attributed to the presence of important pharmacophoric units, such as the imidazole or indole core, respectively. It should be emphasized that the dichloro(1,5-cyclooctadiene)palladium(ii) complex ((COD)PdCl2) containing no amino acid ligands was studied as the reference. The fact that the antibacterial activity of the latter complex appeared to be much lower and the absence of activity of the free ligands provide unambiguous evidence that the presence of a biogenic ligand coordinated to the palladium ion is the main structural factor responsible for this type of activity. It is this combination that determines the optimal lipophilicity(hydrophilicity) of the molecule and ensures the penetration of the latter through the membrane of the pathogenic cell.
Diverse biological activity of heteroleptic complexes Pd49–Pd54 containing acetylpyridine and substituted 2-(2-benzylidenehydrazinyl)pyridines (L49–L54) was investigated (Table 13)[34]. For all ligand–complex pairs, it was clearly established that the chelation of the metal ion leads to the enhancement of antibacterial activity of the ligand by a factor of 3–4. It is noteworthy that the palladium salt Na2PdCl4 did not possess bactericidal properties. The subtle dependence of the antimicrobial activity of the synthesized complexes Pd49–Pd54 on the character of substitution in the benzene ring of the imine ligand was revealed. Complex Pd54 proved to be active against all the tested bacterial strains with MIC values in the range of 30–50 μM. Compound Pd54 exhibited higher activity (MIC was 30 μM against B. subtilis and 40 μM against P. aeruginosa), while complex Pd53 was the most effective against E. coli (MIC = 30 μM).
Coumarins, phenolic compounds, are widely distributed secondary plant metabolites. Coumarin complexes with transition metals exhibit significant biological activity[35]. Numerous PdII complexes with coumarins were synthesized, and their antimicrobial and cytotoxic activity was confirmed. The antibacterial activity of complexes Pd55 and Pd56 based on 4-hydroxycoumarin derivatives was evaluated against 16 strains of microorganisms[36]. Generally, these ligands and their complexes with palladium showed low activity.
Compounds Pd57–Pd59 with furopyran-3,4-dione derivatives were investigated as antimicrobial agents against three bacterial (E. coli, P. aeruginosa and S. aureus) and two fungal strains (С. albicans and С. tropicalis) (Table 14)[37]. Metal complexes Pd57–Pd59 exhibited higher antimicrobial and antifungal activity than the corresponding ligands, which is comparable with the activity of the reference drugs. As regards the nature of the substituent, it should be noted that hydroxycontaining derivative Pd59 showed higher activity. Dechouk et al.[37] suggested that this fact is associated with a higher polarity of this compound due to the presence of the hydroxy group, which provides an interaction (through hydrogen bonding) with the bacterial cell membrane and penetrates the latter. antifungal activity than the corresponding ligands, which is comparable with the activity of the reference drugs. As regards the nature of the substituent, it should be noted that hydroxycontaining derivative Pd59 showed higher activity. Dechouk et al.[37] suggested that this fact is associated with a higher polarity of this compound due to the presence of the hydroxy group, which provides an interaction (through hydrogen bonding) with the bacterial cell membrane and penetrates the latter.
Organic-inorganic complexes Pd60–Pd63 containing the substituted 8-hydroxyquinolinium cation and the tetrachloropalladate anion are effective against 14 strains of microorganisms with MIC values from <1.95 to 250 μg mL–1 (Table 15)[38]. Despite the fact that these metal complexes contain the [PdCl4]2– anion, they exhibit different antimicrobial activity. The observations demonstrated that the cationic part, 8-hydroxyquinolinium derivatives, have a significant effect on the antimicrobial activity. For this reason, the authors tested free ligands L60–L63 against these microorganisms. In the study[38], the complexes were found to have higher activity compared to that of the corresponding ligands, as is often the case. Among the most significant results, noteworthy is the antimicrobial activity of complex Pd61. This complex has MIC <1.95 μg mL–1 in all tests, which is several times higher than the activity of the initial ligand.
Complexes M64, where M = Pd, Cu, Ni, Cd, Rh or Mn, based on imino derivatives of 4-aminoantipyrine (L64) were found to exhibit diverse, including antibacterial, activity[39]. The experiments demonstrated that all metal complexes were more active than the initial ligands against the microorganisms E. coli, K. pneumoniae, P. aeruginosa, S. aureus, S. mutans and C. albicans. However, in most cases the activity was not higher than that of the reference drug. Thus, the diameter of the inhibition zone was in the ranges of 13–41 and 21–30 mm, respectively. An exception is the palladium derivative. For example, initial ligand L64 is inactive against S. mutans; in the presence of complex Pd64, the growth inhibition zone of bacteria is 24 mm, which is comparable with the activity of ampicillin (22 mm).
4-Aminoantipyrine was also used as a scaffold for the synthesis of Schiff bases by the condensation with substituted chromones[40]. The reaction of ligands L65–L67 with palladium chloride affords the corresponding complexes Pd65–Pd67. The antimicrobial activity of these complexes was evaluated against two human bacterial pathogens, such as S. aureus and E. coli. Complexes Pd65–Pd67 showed a larger growth inhibition zone of the bacteria S. aureus (12–21 mm) compared to streptomycin used as the positive control (11 mm). These complexes proved to be inactive against E. сoli.
The screening of a series of complexes M68, where M = Pd, Mn, Co, Ni, Cu, Zn, Fe or Ru, which were synthesized based on the polyfunctional imino derivative of 4-aminoantipyrine (L68), demonstrated that complex Pd68 exhibited the best antifungal activity and the highest antimicrobial activity (Table 16)[41]. It was mentioned that free ligand L68 displayed no activity. The in vitro testing of these compounds was carried out against S. aureus, B. subtilis, E. coli, P. vulgaris, C. albicans and A. fumigates strains.
The biological profile of complexes Pd69–Pd73 and corresponding substituted salicylaldehydes HL69–HL73 as the ligands was determined (Table 17)[42]. The antimicrobial activity was evaluated against two Gram-negative bacteria (Xanthomonas campestris and E. coli) and two Gram-positive bacteria (B. subtilis and S. aureus). All metal complexes proved to be more active than the initial ligands. The best results were obtained for the bromo derivatives both in the group of the ligands (the MIC values of HL69 were in the range from 89 to 179 μM) and complex Pd69 (MIC = 38 μM).
3. Cyclopalladated compounds. Palladium carbene complexes.
Cyclopalladated compounds, including carbene complexes Pd74–Pd76 with a N-donor pyridine moiety, are a special group[43]. In this study, a series of palladium complexes both in the racemic form and as individual enantiomers were synthesized. The latter compounds were obtained by the resolution of a racemic mixture using an additional amino acid ligand as the chiral reagent. The antimicrobial activity of the initial ligands and the corresponding complexes was evaluated against 13 strains of pathogenic microorganisms (Table 18, selected data are given)[43]. This investigation is a rare example of studies, in which not only the structural factors but also stereochemical characteristics of the tested agents are considered. For chiral compounds, it is very important to perform a comparative analysis of the racemic form and individual enantiomers. This issue is confirmed by the results obtained in the study[43]. Racemic complex (R,S)-Pd74 did not exhibit antimicrobial activity at the highest tested concentration (0.5 mM), while the corresponding enantiomer (R)-Pd74 inhibited the growth of the tested microorganisms. For the pair of phenyl-substituted structures of racemate (R,S)-Pd75 and optically pure enantiomer (S)-Pd75, no significant differences were observed because both forms did not show antimicrobial activity at the highest tested concentration (0.5 mM).
N-Heterocyclic carbene (NHC) complexes are promising compounds for searching for new pharmacological agents[44-48]. Among different NHC systems, air-stable and convenient-tohandle complexes stabilized by pyridine, so-called PEPPSI complexes (PEPPSI stands for pyridine-enhanced precatalyst preparation, stabilization and initiation), have attracted great attention.
In order to evaluate the effect of structural factors on the biological activity of the PEPPSI-type complexes, a large library of benzimidazolium salts and related palladium carbene complexes was synthesized[45]. The following two groups of complexes should be distinguished: one group (Pd77a–i) contains pyridine as the stabilizing ligand; another group (Pd78a–h) contains triphenylphosphine (Table 19). In each group, the number and positions of alkyl substituents in aromatic rings of the benzimidazole ligand were varied. A broad biological activity profile of the synthesized compounds was investigated. The antimicrobial activity was evaluated using E. coli, MRSA and C. albicans strains as the tested microorganisms. Eight compounds exhibited higher antibacterial activity against E. coli compared to that of tetracycline. Complexes Pd77g and Pd78f were found to be the most active (the inhibition zone was 26.3 mm at a concentration of the compounds equal to 50 μg per disc). Compounds Pd77f, Pd78f and Pd78c showed higher activity against MRSA compared to the reference drug (the inhibition zones were 28.5, 28.0 and 27.0 mm, respectively). Complexes Pd77b, Pd77g and Pd78e exhibited the best antifungal activity against the yeast-like fungi C. albicans with the inhibition zone of 32.0, 29.5 and 29.0 mm, respectively. Generally, the triphenylphosphine complexes were more active in most of the tests (the ratio of the active compounds with triphenylphosphine and pyridine ligands was 18:9). However, these results did not allow a definite conclusion about the effect of the character of substitution in the benzene ring of the ligand on the antibacterial activity.
As a continuation of these studies, a new series of benzimidazolium salts HL79a–e and related palladium carbene complexes Pd79a–e were synthesized (Table 20)[46]. The antimicrobial activity of these compounds was evaluated against the strains M. luteus LB14110, Listeria monocytogenes ATCC 19117, S. typhimurium ATCC 14028 and S. aureus ATCC 6538. Most of ligands HL79a–e showed low activity against all the tested bacterial and fungal strains. Complexes Pd79a–e displayed moderate selective activity, except for compound Pd79с, which showed high inhibitory activity against all the tested strains. In the study[45], this fact was not explained. It can be suggested that the presence of the maximum number of nonpolar methyl substituents on the aromatic ring in compound Pd79с provides the required hydrophilic lipophilic balance for the penetration into the microbial cells.
In the studies[47, 48], a number of new related PdII carbene complexes were investigated. The antimicrobial activity of compounds Pd80a–e was evaluated against the same range of pathogenic microorganisms. The comparative results given in Table 20 demonstrate that these structural changes did not lead to a significant improvement of the activity.
Binuclear palladacycles can be used to synthesize mononuclear mixed-ligand complexes[49]. Compounds Pd81 and Pd82 thus synthesized were tested as antibiotics against E. coli. The distinguishing feature of these structures is the presence of substituents of different nature. Thus, complex Pd81 contains an OH group, while its analogue Pd82 contains a remote amino group as a quaternary salt, thereby providing better solubility of Pd82 in an aqueous medium and allowing the use of more concentrated solutions in the testing. It was found that the palladium complexes exhibited satisfactory antibacterial activity, which is higher than that of the initial organic ligands. At a concentration of 0.1 mM, complex Pd81 caused 50% inhibition of complex-forming units (compared to the control). The same result with the use of compound Pd82 was achieved at a concentration of 0.6 mm; the 70% inhibition, at 1 mM.
4. Palladium complexes with sulfur-containing ligands.
Palladium(ii) complexes with sulfur-containing ligands, including S-donor ligands, are extensively investigated as antimicrobial agents. A large series of PdII, PtII and ZnII complexes of this structural type were synthesized by the research group headed by Al-Janabi[50-53].
Polydentate diazene ligand HL83 allows the synthesis of different types of palladium(ii) and platinum(ii) complexes depending on the reaction conditions[50]. In a neutral medium, the reaction affords compounds Pd83a and Pt83a; in an alkaline medium, dimers Pd83b and Pt83b are produced. The evaluation of their antibacterial activity against S. aureus, E. coli, K. pneumoniae and P. aeruginosa strains demonstrated that palladium complexes Pd83a and Pd83b exhibited the highest activity (Table 21). However, all the tested compounds were less effective than gentamicin used as the reference drug.
This research group also tested the antifungal activity of complexes Pd84–Pd86 with thiol ligands against two types of fungal strains of C. albicans and A. niger (Table 22)[51]. These complexes are distinguished by the structure of the second chelating N,N-donor ligand. Generally, these compounds did not exhibit satisfactory antifungal activity. However, it is worth mentioning the following interesting issue. Complex Pd85 exhibited abnormally high inhibitory activity against the fungi C. albicans comparable with the activity of fluconazole used as the reference drug.
The study[52] is a continuation of the above investigations. In this study, a series of mixed-ligand dithiocarbamate complexes of palladium (Pd87–Pd90) and zinc (Zn87, Zn88) were synthesized. The antimicrobial activity of these compounds was evaluated against three types of pathogenic bacteria (E. coli, S. aureus and S. pyogenes) and two types of fungi (C. albicans and A. niger) (Table 23). First of all, it should be noted that the dithiocarbamate ligand, sodium piperidine dithiocarbamate (NaPipDT), used for the comparison has low activity. Based on the analysis of the structure–property relationship, the authors concluded that the specificity of antibacterial activity does not depend on the nature of metal. Thus, the related Pd and Zn complexes (Pd87 and Zn87, Pd88 and Zn88) exhibited comparable activity against the whole series of the tested strains. The complexes possessed moderate to good activity compared to the standard antimicrobial drug streptomycin. Complexes Pd89 and Pd90 with the phosphine ligand appeared to be more effective antimicrobial agents than the other complexes and the free ligand. It is worth noting that complex Pd90 containing, unlike compound Pd89, the oxidized sulfamide moiety showed higher antibacterial activity than streptomycin and higher antifungal activity compared to that of fluconazole.
Dithiocarbamate ligands derived from the primary amines N-phenylaniline, 4-methylaniline and 4-ethylaniline were used to prepare NiII, PdII and PtII complexes[54]. Table 24 gives selected data on the activity of complexes Pd91–Pd93. Unlike the previous study, Bobinihi et al. [54] proposed the bidentate S,S-chelating mode of coordination of the dithiocarbamate ion to the metal cation. The antimicrobial screening demonstrated that complexes Pd91–Pd93 exhibited moderate activity against Gram-negative (E. coli, K. pneumoniae and P. aeruginosa) and Gram-positive bacteria (Bacillus cereus and S. aureus) and against fungi (C. albicans and A. flavus) at a concentration of 50 μg mL–1. Ethyl-substituted dithiocarbamate complex Pd93 showed better antimicrobial activity compared to that of the unsubstituted (Pd91) and methyl-substituted (Pd92) analogues.
Thiocarbamate complexes Ni94, Pd94 and Pt94 were synthesized and characterized (Table 25)[55]. The tested S. aureus, B. cereus, P. aeruginosa, E. coli and C. albicans strains proved to be resistant to the free ligand. The metal complexes exhibited moderate antimicrobial activity comparable with the activity of the reference antimicrobial drugs.
Complexes Pd95a,b and Pt95a,b were synthesized by the reaction of Na2PdCl4 or K2PtCl4 with 4-methylidene-3-phenyl3,4-dihydroquinazoline-2(1H)-thione (HL95). In the presence of an alkali, the reaction proceeds through the deprotonation to give complexes Pd95b and Pt95b; in the absence of an alkali, the reaction affords complexes Pd95a and Pt95a[53]. The antibacterial activity of these compounds was evaluated against five pathogenic bacteria: P. aeruginosa, K. pneumoniae, E. coli, S. epidermidis and S. aureus (Table 26). It was found that all metal complexes were more effective than the free ligand. It should be noted that the palladium compounds were slightly less effective than the platinum analogues. Generally, the activity of these complexes is similar to the activity of tetracycline used as the reference drug.
A series of mononuclear complexes Pd96–Pd101 with pyrazolylsulfonamide ligands were investigated in the study[56]. The antibacterial activity was evaluated against Gram-negative (Citrobacter freundii, E. coli, S. typhi, P. mirabilis, Vibra cholerae, K. pnuemoniae) and Gram-positive bacteria (S. aureus, E. faecalis, S. epidermidis) (Table 27). The MIC values demonstrated that the tested compounds are more active against Gram-negative bacteria compared to Gram-positive bacteria. Note once again that, in general, the palladium complexes possess higher antibacterial activity than the corresponding ligands.
Complexes Pd102 and Ru102 were synthesized using an appropriate polydentatс ligand belonging to Schiff bases[57]. The antibacterial activity of ligand L102 and its metal complexes was evaluated against five bacterial strains: MRSA ATCC 43300, S. aureus ATCC 25923, P. aeruginosa ATCC 278530, M. luteus and E. coli ATCC 25922. It was found that free ligand L102 was inactive against all the tested bacterial strains. Complex Pd102 exhibited activity only against methicillinresistant Staphylococcus aureus (the diameter of the inhibition zone was 19 mm at a concentration of 10 mg mL–1) and methicillin-sensitive S. aureus (16 mm). A significant difference in the bactericidal activity against the E. coli strain was observed between complexes Ru102 (20 mm) and Pd102 (0 mm)
The applied aspect of using of these metal complexes for the analytical detection of free cholesterol in human blood was investigated[58]. Fluorometric measurements showed significant fluorescence quenching induced by the reaction of complex Pd102 with cholesterol, which is indicative of a sensitive fluorometric response, allowing the detection of cholesterol. The detection limit of cholesterol using complex Pd102 at a concentration of 4.6 μM was much lower than the detection limit achieved using other known sensors in the cholesterol concentration range of 0–5 mM.
A comparative analysis of the antimicrobial activity of chelate complexes M103, where M = VO, Zr, Pd, Pt or U, with 2-cyano-2-[(2-nitrophenyl)hydrazono]acetamide (L103) was performed (Table 28)[58]. The bactericidal effect was evaluated against two Gram-positive (S. aureus K1 and B. subtilis K22) and two Gram-negative bacteria (E. coli K32 and P. aeruginosa SW1) compared to the positive control (ampicillin, amoxicillin and cephalexin). Complex Pd103 exhibited the highest antibacterial activity. However, the fungicidal activity of this compound against two pathogenic fungi (A. flavus and A. fumigatus) proved to be lower than that of complexes Zr103 and Pt103. The activity of initial ligand L103 was much lower than that of the metal complexes in all tests. It is worth noting that the structures of the complexes presented in the original study[58] are doubtful.
A comprehensive theoretical and physicochemical study of palladium complex Pd104 with Schiff base HL104, which was synthesized by the condensation of 8-acetyl-7-hydroxy-4- methylcoumarin with sulfaclozine (sulfachloropyrazine), was performed[59]. This is yet another example of a multimodal ligand with pharmacophoric moieties. Sulfaclozine is an effective sulfonamide derivative with antibacterial activity, which is widely used for the treatment of various poultry diseases. It was found that the antibacterial activity of complex Pd104 against S. faecalis, E. сoli and Neisseria gonorrhoeae strains is higher than the activity of initial ligand HL104. Complex Pd104 proved to be generally less active against a series of pathogenic strains (B. subtilis, S. aureus, S. faecalis, E. coli, N. gonorrhoeae, P. aeruginosa) compared to complex Zn104 described in the same study but is more active than complex Cu104.
The solubility of these agents in aqueous solutions is an important issue. Metal complexes Pd105 and Pd106 were synthesized using water-soluble sulfonate ligands[60, 61]. The antimicrobial potential of ligand HL105, which was prepared based on isatin, and corresponding complex Pd105 was evaluated against three known bacterial strains of E. coli, S. marcescens and S. aureus; the antifungal activity, against A. flavus, C. albicans and T. rubrum strains[60]. The MIC values of ligand HL105 varied in the range of 6.50–8.05 μM; of complex Pd105, in the range of 1.75–2.20 μM. In the case of the fungal strains, the MIC values were 6.65–7.85 μM (for HL105) and 2.05–2.50 μM (for Pd105). Therefore, complex Pd105 proved to be more active than the corresponding free ligand.
Related ligand HL106 was synthesized based on picolinic acid hydrazide[61] and was used to prepare a series of metal complexes M106, where M = Co, Fe, Hg or Pd. These complexes were subjected to antimicrobial screening against the following microorganisms: S. aureus, B. subtilis, E. coli, P. aeruginosa and C. albicans. Unlike complex Pd105, compound Pd106 did not exhibit sufficiently high activity against the tested strains. Picolinic acid hydrazide was used also in the study[62] to synthesize thiosemicarabazides Н2L107–Н2L109 and heterocyclic complexes Pd107–Pd109 and Pt107–Pt109. Analyzing the results of antimicrobial screening of these ligands and their metal complexes, it can be stated that only two results are worth discussing. Thus, complexes Pd108 and Pt108 containing a cyclohexyl substituent possessed high antibacterial activity against B. subtilis and Lactobacillus fermentum. The MIC values of these compounds were lower than 16 μg mL–1, which is smaller than the value of 32 μg mL–1 for ampicillin taken as the control.
Abu-Dief and co-workers[63-65] tested thiazolopyrazole complexes of PdII, CuII and FeIII as antimicrobial agents. The antibacterial screening was carried out against the bacteria M. luteus, E. coli and S. marcescens; the fungicidal activity, against the fungi F. oxysporum, G. candidum and A. flavus. In all tests, complexes Pd110–Pd112 proved to be more effective than the corresponding ligands and complexes Cu110–Cu112 and Fe110–Fe112 (Table 29, selected data are given).
As mentioned by de Lacerda et al.,[66] the resistance of the zoo bacterium Campylobacter jejuni, which causes foodborne gastroenteritis, to clinical antimicrobial agents is a challenging problem. The antibacterial activity of two new complexes (Pd113 and Pt113) was evaluated against three resistant C. jejuni strains. The in vitro antibacterial activity of these complexes was investigated in isolation and synergistically with ciprofloxacin (CIP) and erythromycin (ERY). Complex Pd113 proved to be ineffective, while its analogue Pt113 gave an interesting synergistic effect with CIP. The grouped analysis of the mean MIC values showed that complex Pt113 was effective both alone (MIC = 21.4±10.6 mg L–1) and in synergy with the antimicrobial drugs (in the presence of CIP, MIC = 0.9±0.5 mg L–1; in the presence of ERY, MIC = 21.4±10.6 mg L–1) compared to the assays, in which only these antibiotics were used (MIC = 32 mg L–1).
The antimicrobial activity of complexes Pd114 and Pd115 with aminothiazole ligands was evaluated against a wide range of pathogenic microorganisms (Table 30, selected data are given)[67]. The experiment included eight bacterial strains (four standard strains and four clinical isolates) and three types of yeast. In most cases, the bactericidal activity of complexes Pd114 and Pd115 was higher than that of the corresponding ligands L114 and L115. The tested complexes exhibited better activity against Gram-positive bacteria (standard and clinical strains of S. aureus and B. subtilis). The effect on Gram-negative bacteria was low. These compounds exhibited moderate antifungal activity. In this case, ligands L114 and L115 showed higher activity than the complexes. The yeast strain of Rhodotorula mucilaginosa was the most sensitive within the positive control (fluconazole).
Complexes Pd116, Ni116 and Zn116 were synthesized by the coordination of the corresponding metals with sulfurcontaining triazole ligand L116 (Table 31)[68]. The evaluation of antimicrobial activity of these compounds against bacterial (S. aureus, E. сoli) and fungal strains (C. albicans, A. flavus) demonstrated that the bactericidal activation of the ligand occurs only upon coordination to palladium (the diameter of the inhibition zone varied from 6–8 to 11–12 mm). Besides, only complex Pd116 exhibited antifungal activity comparable with the activity of miconazole used as the reference drug.
Aminotriazole and oxodiazole thiones are close analogues of the above ligands. These ligands were used to synthesize mononuclear (Pd117a and Pd118a) and binuclear (Pd117b and Pd118b) palladium(ii) complexes (Table 32)[69]. All the synthesized compounds were tested as antimicrobial agents against eight bacterial strains (S. aureus ATCC 25923, S. aureus (laboratory isolate), E. faecalis ATCC 29212, E. coli ATCC 25922, P. aeruginosa ATCC 27853) and three fungi (A. niger, C. albicans, Trichosporon). No significant antifungal activity was observed in this series. The patterns of changes in the antibacterial activity are significantly differen for the aminotriazole and oxodiazole derivatives. Thus, aminotriazole sulfone HL117 exhibited high bactericidal activity against the whole range of strains (the diameter of the growth inhibition zone of bacteria was 15–34 mm), while corresponding complexes Pd117a,b proved to be inactive. On the contrary, ligand HL118 was inactive against Gram-negative bacteria (E. coli, P. аeruginosa), while its complex Pd118b inhibited the growth of bacteria (the diameters were 7 and 11 mm, respectively).
The imine [(4-isopropoxybenzylidene)amino]-5-propyl-3- sulfanyl-4H-1,2,4-triazole (HL119) was synthesized based on aminotriazole thione and was used to prepare a series of cobalt(ii), nickel(ii), zinc(ii), copper(ii) and platinum(ii) complexes[70]. Depending on the ligand:metal molar ratio, the coordination results in the formation of mono- and bischelate complexes (e.g., Pd119a and Pd119b). The antibacterial activity of these compounds was tested against the standard strains S. aureus MTCC 96, S. pyogenes MTCC 442, E. coli MTCC 443 and P. aeruginosa MTCC 1688 (Table 33). The tested compounds showed moderate activity (MIC = 62.5–250 μg mL–1); however, the lead compound was not found. The dependence of the activity on the nature of metal and the type of the complex was also not revealed. For compounds Pd119a and Pd119b, only the effective bactericidal activity against Pseudomonas aeruginosa and Streptococcus pyogenes was observed (MIC = 62.5 μg mL–1), which is comparable with the activity of the clinical drug, the antibiotic chloramphenicol (MIC = 50 μg mL–1). For comparison, note that the MIC value of free imine HL119 was in the range of 100–125 μg mL–1. Unlike the antibacterial activity, the antifungal effect of the tested metal complexes against C. albicans, A. niger and A. clavatus strains was low (MIC = 250–1000 μg mL–1), which is lower than that of nystatin (100 μg mL–1). Nevertheless, it is clearly seen that the fungicidal activity of the bischelate complexes is higher than that of the monochelate analogues. For example, compound Pd119a is almost inactive (MIC = 1000 μg mL–1 and higher); the MIC value of complex Pd119b is in the range of 250–1000 μg mL–1 against all the tested pathogenic fungi.
As it was mentioned many times above, and efficient and promising approach to tackle the problem of resistance of clinical drugs is based on their modification through complexation with metal ions. An example of this approach is the study[71], in which complexes Pd120–Pd122 with sulfamethoxazole imines were synthesized and characterized. The evaluation of the antibacterial activity against the microorganisms E. coli, K. pneumoni, S. aureus and B. subtilis showed that corresponding ligands L120–L122 exhibited moderate activity, which is, in some cases, enhanced upon their coordination to metal ions (Table 34). For example, the MIC value of ligand L120 for Staphylococcus aureus is 200 μg mL–1, while this value for corresponding complex Pd120 is 50 μg mL–1. The results of this study demonstrate that sulfamethoxazole derivatives and their metal complexes possess low activity against the pathogenic fungi C. albicans and A. niger.
Sulfamethoxazole was used also to synthesize ligand L123 and related complexes Pd123 and Pt123 (Table 34)[72]. Unfortunately, it is impossible to compare the results of these two studies (see [71, 72]) because the antimicrobial activity of the synthesized compounds was expressed in different units. Nevertheless, it is worth noting that ligand L123 and corresponding complex Pd123 exhibited good antibacterial activity against E. coli (the diameter of the inhibition zone was 16 mm, the concentration was not reported). Complexes Pd123 and Pt123 possessed higher activity against the bacteria S. aureus (15 mm) compared to free ligand L123 (10 mm). The free ligand was inactive against P. aeruginosa, whereas complexes Pd123 and Pt125 inhibited this bacterial strain, compound Pd123 exhibiting higher inhibitory activity than complex Pt123 (the diameters of the inhibition zone were 15 and 10 mm, respectively). Notably, complex Pd123 was more active against the bacteria P. aeruginosa compared to the standard drug ampicillin.
Original macrocyclic ligand L124 and complex Pd124 were synthesized and characterized[73]. The antimicrobial activity of this pair of compounds was evaluated against S. aureus. It was found that the coordination to the metal causes a decrease in the activity compared to free ligand L124 (MIC = 6.25 μg mL–1 for the ligand; MIC = 25 μg mL–1 for Pd124). The ligand and the complex had equal antifungal activity against the yeast C. albicans (MIC = 6.25 μg mL–1).
A wide screening of complexes Pd125–Pd127 with thiazole azomethine ligands for antimicrobial activity was performed (Table 35)[74]. In this study, 16 bacterial strains and two fungal strains were used. All compounds proved to be the most effective against Gram-positive bacteria, particularly against B. megaterium. Generally, complexes Pd125–Pd127 exhibited higher antibacterial and antifungal activity compared to corresponding ligands HL125–HL127. The best results were observed for compound Pd126 containing an amine substituent. For example, the activity of this compound against the microorganisms S. aureus is comparable with that of the reference drug vancomycin.
Salicylidene derivatives of thiosemicarbazones were used as polydentate ligands to synthesize chelate complexes Pd128–Pd131 having N,O,S-coordination mode[75]. The antimicrobial activity of these ligands and palladium(ii) complexes was evaluated against a panel of pathogenic bacterial and fungal strains (Table 36). It was found that thiosemicarbazone ligands HL129–HL131 did not exhibit inhibitory activity against this panel of strains, except for the fungi C. tropicalis. The test cultures of Gram-negative bacteria (P. aeruginosa, E. coli, K. pneumoniae, P. mirabilis) and S. aureus proved to be resistant to all these compounds. Complexes Pd128 and Pd129 with thioalkylated ligands possessed significant activity against E. faecalis and S. epidermidis strains, whereas thione complexes Pd130 and Pd131 did not inhibit the growth of these bacteria. All complexes Pd128–Pd131 displayed fungicidal activity. For example, complex Pd128 appeared to be more active against C. albicans (MIC = 3.17 μg mL–1) than the reference drug clotrimazole (MIC = 4.9 μg mL–1).
Selenium is an essential natural microelement. Therefore, it is reasonable to introduce this heteroatom into potential pharmaceticals[76]. Chiral selenium-containing imines HL132, HL133 and related complexes Pd132, Pd133 were tested for biological activity. The antimicrobial activity was evaluated against the microorganisms K. aerogenes, E. coli, P. desmolyticum, S. aureus and C. albicans using the clinical drugs ciprofloxacin and fluconazole as positive controls. However, all the tested compounds exhibited very low activity. Thus, the diameter of the growth inhibition zone of the tested microorganisms for the reference drugs (ciprofloxacin, fluconazole) was in the range of 11–14 mm, whereas this parameter for complexes Pd132–d133 was smaller than 5 mm even at a concentration of 10 mg mL–1.
The cycloaddition of aryl-substituted benzoic acid hydrazides to CS2 afforded a series of oxadiazolothiones L134–L139, which were used as ligands to synthesize complexes Pd134–Pd139[77]. Hydrazides L140–L146 and their complexes Pd140–Pd146 were also investigated in order to perform a comparative analysis and reveal the structure–property relationships. The antibacterial and antifungal activity of two types of complexes, with the N,S-chelating thio ligand (Pd134–Pd139) and the monodentate N-coordinated hydrazide ligand (Pd140–Pd146), was evaluated. The structural diversity of the compounds was ensured by the nature and positions of substituents in the aromatic ring of the ligands. The antimicrobial potential of palladium complexes Pd134–Pd146 and free ligands L134–L146 was evaluated against a wide range of pathogenic bacteria (M. luteus, S. aureus, MRSA, E. coli, S. typhi, P. aeruginosa) and fungi (A. niger, C. albicans, A. flavus, Microsporum gypseum, Mucor, Penicillium, Saccharomyces, T. mentagrophytes) (Table 37, selected data are given). The following distinguishing features were observed in the group of hydrazide ligands and complexes Pd140–Pd146: in most tests, the ligands are less active than the corresponding palladium(II) complexes; the powerful antibacterial agent Pd142 (R = 4-NO2) was found, which provided the inhibition zone with a diameter of 11–16 mm for four of the six tested bacterial strains (the activity against the bacteria P. aeruginosa and MRSA is comparable with that of ampicillin). Unlike the hydrazide compounds, oxadiazolothiones L134–L139 exhibited stronger antibacterial activity than the corresponding palladium complexes against most of bacterial strains. Compounds Pd134–Pd139 are also weaker antibacterial agents than related complexes Pd140–Pd146. This fact was attributed to the higher structural stability of the bidentate chelate complexes. In the series of complexes Pd134–Pd139, compounds Pd138 (R = 2-Cl) and Pd135 (R = 4-NO2) showed the highest antibacterial potential against strains of P. aeruginosa (the diameter of the inhibition zone was 12 mm) and E. coli (10 mm). The effect of the position of the substituent on the antibacterial activity of compounds Pd134–Pd139 and Pd140–Pd146 is almost the same. Thus, compounds containing electronwithdrawing substituents in ortho or para positions possess the highest activity. As opposed to antibacterial activity, such patterns were not observed for antifungal activity. 4-Pyridyl derivatives Pd139 and Pd146 are rather active fungicidal agents.
5. Palladium complexes for antimicrobial photodynamic therapy
Photodynamic therapy (PDT) is an efficient method used in clinical practice to overcome the antibiotic resistance of known pathogenic microorganisms. This method is based on the combined action of a photosensitizer, oxygen and light of a particular wavelength. The reaction affords singlet oxygen and other reactive oxygen species, which can inactivate pathogenic cells. Porphyrin complexes of transition metals, in particular palladium, are extensively studied as photosensitizers[78-82]. It is worth noting that the palladium-containing agent padeliporfin (Pd147) is currently used for PDT[83].
In the studies[78, 79], phthalocyanine complex Pd148a with four peripheral N-methylpyridiniomethoxy substituents was found to be effective against the heterotrophic Gram-negative rod-shaped bacterium Aeromonas hydrophila. Compound Pd148a was synthesized and characterized as a mixture of structural isomers with different arrangement of cationic substituents on the aromatic ring. Strains of the heterotrophic Gram-negative rod-shaped bacterium A. hydrophila, resistant (R) and sensitive (S) to antibiotics, were investigated. Peripherally substituted complex Pd148a showed complete photoinactivation at a concentration of 8 μM for the sensitive A. hydrophila strain. At a concentration of 20 μM, this compound exhibited dark toxicity against both tested strains (S and R). At lower concentrations (<2 μM), Pd148a did not show phototoxicity against either S or R strains.
In the subsequent study[79], these authors applied this procedure to clinical isolates of the Gram-negative bacterium F. hydatis, namely multiresistant (R) and sensitive (S) strains. Different structural isomers of palladium(ii) phthalocyanine complexes containing one of N-methylpyridiniomethoxy groups in the meta (Pd148a) or ortho position (Pd148b) were used as bacterial growth inhibitors. It was found that dark toxicity was absent in all tests. Only complex Pd148b was shown to have relatively high antibacterial photoefficiency; lower efficiency was observed for its analogue Pd148a. It was found that the photodynamic response was similar regardless of the resistance of the bacterial strain; it depended only on the photosensitizing ability of the applied phthalocyanine complex.
Phthalocyanine L149 containing nipagin-functionalized substituents and its metal complexes Pd149 and Zn149 were investigated as photosensitizers for antimicrobial photodynamic therapy[80]. The photoactivity of compounds L149, Pd149 and Zn149 encapsulated into a liposomal formulation was tested against a wide range of microorganisms, including the bacteria MRSA and E. coli (ESBL+), the fungi C. albicans (fluconazoleresistant) and C. auris and the dermatophytes Т. mentagrophytes and Т. rubrum. The first thing to note is that, in the absence of irradiation, no growth inhibition was observed across all the tested strains or compounds even at a concentration two times higher than that used in the experiments with irradiation. There was a clear relationship between the presence of ZnII or PdII in the macrocyclic core and their phototoxicity against the tested microorganisms. Free ligand L149 appeared to be the least active, whereas derivative Pd149 exhibited significantly better bactericidal activity compared to its zinc analogue Zn149.
Porphyrins with an extended π system were synthesized and used to prepare corresponding complexes Zn150 and Pd150[82]. The electronic modification of the structures makes it possible to significantly extend the absorption spectrum and, correspondingly, the field of application of these agents. The antibacterial activity of metalloporphyrins Zn150 and Pd150 was evaluated against two resistant strains, S. aureus 3150/12 and B. subtilis DB104. Complex Pd150 showed significantly higher activity than its zinc analogue Zn150. For example, in the presence of compound Pd150, bacterial colonies S. aureus 3150/12 were not found under continuous irradiation at a dose of 18 J cm–2 for 60 min.
Porphyrins containing peripheral positively charged PtII (or PdII) dipyridyl complex moieties are of biological and photochemical interest[84-86]. As mentioned in these studies, such compounds are the best generators of ROS. Two isomeric PdII porphyrins, Pd151 and Pd152, were used in experiments on the photoactivation of E. coli, K. pneumoniae, P. aeruginosa and S. aureus bacterial cells, (Table 38)[84]. It was found that complexes Pd151 and Pd152 did not exhibit antimicrobial activity without exposure to light. Under irradiation, the death of the cells of all the tested microorganisms was observed. The study demonstrated that porphyrin Pd151 containing a positively charged group in the meta position was the most efficient photosensitizers.
In the subsequent studies[85, 86], this team of Brazilian researchers investigated this type of complexes for PDT against such pathogenic microorganisms as mycobacteria and fungal dermatophytes. In the latter case, related platinum(ii) complexes were used for comparison. The latter complexes appeared to be more efficient photosensitizers than the palladium(ii) complexes.
Another example of active photosensitizers is PdII complexes with meso-substituted porphyrins, which were prepared both in the neutral amino form (Pd153) and as quaternary ammonium salts (Pd154)[87]. Chitosan conjugates of these compounds were also synthesized. The in vitro photodynamic antibacterial activity of these agents was evaluated against S. aureus. The highest activity was observed for positively charged porphyrin Pd154 and its chitosan conjugate. Almost complete degradation of bacterial cells was achieved after 15–30 min irradiation time at a photosensitizer concentration of 0.5 μm. This result was attributed to the fact that the presence of positively charged centres in palladium porphyrin provides better cell accessibility of this compound.
Derivatives of coumarin (diferoylmethane), a natural β-diphenolic diketone pigment, are highly promising photosensitizers[88]. The coordination to metal ions makes it possible to significantly change the photophysical properties of these ligands. Heteroleptic complexes Cu155 and Pd155 with an additional phenanthroline ligand were synthesized. The in vitro antimicrobial photoactivity of these compounds was evaluated against fungi (C. albicans, Sporothrix brasiliensis, M. canis, M. gypseum and Epidermophyton floccosum) and protozoa (Leishmania amazonensis), which cause clinically essential surface skin diseases in humans. The results obtained in this study demonstrate that metal coumarin complexes are promising candidates for PDT. In particular, it was found that the antifungal activity of complexes Cu155 and Pd155 against S. brasiliensis strains is enhanced when using light instead of dark testing (MIC is 10 μm vs. 100 and 25 μm).
6. Palladium complexes with terpene ligands
Palladium complexes with terpene ligands are in the focus of our research[89]. A series of diverse PdII complexes containing pinaneor bornane-type ligands were evaluated for antimicrobial activity. Below, two compounds belonging to cyclopalladated benzylamines (Pd156 and Pd157) and two chelate complexes (Pd158 and Pd159) with terpene derivatives of ethylenediamine are given as examples. Biological tests were performed in vitro in collaboration with the not-for-profit initiative CO-ADD. The activity of the compounds was evaluated based on the inhibition of cell growth of five types of bacteria (S. aureus, P. aeruginosa, K. pneumoniae, A. baumannii, E. coli) and two types of fungi (C. albicans, C. neoformans). These types of commonly occurring bacteria and fungi have numerous mechanisms of resistance to a series of clinical drugs, making the search for new bactericidal and fungicidal agents against them a challenging problem.
According to these studies, palladium(ii) complexes with different structures showed high selective inhibitory activity against a series of bacterial strains (Table 39). All the tested compounds were found to be inactive against the Gram-negative bacteria P. aeruginosa, K. pneumoniae, A. baumannii and E. coli. Complexes Pd156 and Pd157 belonging to cyclometallated compounds exhibited high inhibitory activity for Gram-positive bacteria, e.g., S. aureus. Palladacycle Pd156 with the MIC value of 1 μg mL–1, which is comparable with the activity of the standard antibiotic vancomycin, was a lead compound. According to the tests, complexes Pd156–Pd159 exhibited high antifungal activity against C. аlbicans and C. neoformans strains. The MIC values were lower than 16 μg mL–1; in some cases, <0.25 μg mL–1. There was no clear structure–property relationship.
To evaluate the cell cytotoxicity of complexes Pd156–Pd159, the СС50 parameter was determined using human embryonic kidney cells HEK293 as the test system. The hemolytic activity (HC10) of the tested agents against human red blood cells (RBC) was assessed. All samples with the СС50 and HC10 values larger than the maximum tested concentration (32 μg mL–1) were classified as nontoxic. Only complex Pd159 met these conditions. This result suggests that the toxicity of palladium coordination compounds depends on the ligand environment of the metal ion and, therefore, can be specifically decreased.
7. Conclusions
An analysis of the literature data allows us to definitely conclude that palladium(ii) complexes possess a high pharmacological potential. Palladium-containing agents have attracted interest mainly as anticancer agents (by analogy with cisplatin), but the evaluation of their antimicrobial activity also has good prospects. This review presents numerous examples of high antibacterial and fungicidal activity of PdII complexes, which is higher than the activity of antibiotics and antifungal drugs widely used in clinical practice. It is worth noting the great structural diversity of the characterized palladium-based compounds. The review gives original, sometimes quite controversial, structural formula of metal complexes. The detailed discussion of synthetic issues is beyond the scope of this review. All relevant literature can be found in the corresponding references to original papers.
In many studies included in this review, the biological profile of palladium(ii) complexes was determined. We selected the most significant results of research on the antibacterial and antifungal activity of these complexes. An analysis of the literature data suggests that even very fine structural changes can lead to significant changes in the biological activity of PdII complexes. It is virtually impossible to select the most promising type of ligands for the design of effective palladium-based antimicrobial agents. A promising approach is based on the use of multifunctional compounds with known biological activity, including those used in clinical practice, as ligands. It can only definitely be stated that metal complexes are more active than the corresponding ligands (this fact was experimentally confirmed in more than 90% publications).
It is worth noting that many publications included theoretical (in silico) studies of the biological activity of PdII complexes[20, 21, 24, 25, 36, 37, 50, 57, 61, 63, 65, 71, 72, 77, 27-30, 52-55]. The molecular docking is a fashionable approach, which allows the identification of the active site for interaction (or the absence of this site) of the tested compound with biomolecules. We did not discuss these results because there is generally no clear correlation between the experimental data on the antimicrobial activity of metal complexes and theoretical calculations.
The elucidation of the mechanism of antibacterial and cytotoxic activity of metal-containing agents is a challenging problem. In a number of studies, interactions of these agents with such biomolecules as DNA or proteins were investigated by photometric or viscosimetric methods[13, 31, 34, 38, 40, 64, 65, 67, 69, 15-18, 23-29]. The available data do not allow one to make a general conclusion although the interaction of metal complexes with biomolecules is clearly detected.
The facts that, on the one hand, many PdII complexes with high antimicrobial activity were fond and, on the other hand, there are no clear structure–property relationships suggest that the high-throughput screening of libraries of different derivatives is the most promising method for searching for effective antibiotics and antimicrobial drugs. Chemists synthesized a large number of new compounds, which are generally difficult to characterize by biological assays. These issues are usually solved individually, which is inefficient. In our opinion, there is a need to establish an all-Russian centre for biological tests of different classes of chemical compounds, in particular promising metal-containing agents.
In concluding this review, we cannot say about such an important form of palladium-containing antimicrobial agents as biogenic palladium nanoparticles (Pd-NPs). This group of compounds is not included in the present review devoted to palladium complexes with organic ligands. Nevertheless, taking into account great prospects of biomedical applications of Pd-NPs, let us refer to a number of reviews[90-97] focused on this issue. In the past years, the scientific community was interested in the biogenic synthesis of Pd-NPs according to the principles of green chemistry. This is a facile, fast, cost-efficient and environmentally friendly method for the preparation of NPs based on the naturally occurring biomolecules or metabolites of different plants and organisms as reducing and stabilizing agents. It was demonstrated that biogenic processes allow for a very high level of control over such important properties of PdNPs as the size and shape.
We hope that the data considered in this review will be interesting and useful for researchers dealing with investgations in medicinal chemistry and chemistry of metal complexes.
List of abbreviations and designations
CC50 — concentration of the agent at which the cell growth is inhibited by 50%,
CIP — ciprofloxacin,
CO-ADD — Community for Open Antimicrobial Drug Discovery,
Су — cyclohexyl,
ERY — erythromycin,
HC10 — hemolytic concentration of the agent corresponding to 10% hemolysis,
HEK293 — human embryonic kidney cells,
MBC — minimum bactericidal concentration,
MIC — minimum inhibitory concentration,
NHC — N-heterocyclic carbene complexes,
Pd-NP — palladium nanoparticles,
PDT — photodynamic therapy,
PEPPSI — pyridine-enhanced precatalyst preparation, stabilization and initiation,
PipDT — piperidine dithiocarbamate,
RBC — human red blood cells,
ROS — reactive oxygen species.
Full names of microorganisms:††
A. baumannii — Actinetobacter baumannii (–),
A. clavatus — Aspergillus clavatus (f ),
A. flavus — Aspergillus flavus (f ),
A. fumigatus — Aspergillus fumigatus (f ),
A. niger — Aspergillus niger (f ),
A. hydrophila — Aeromonas hydrophila (–),
B. animalis subsp. lactis — Bifidobacterium animalis subsp. Lactis,
B. brevis — Bacillus brevis (+),
B. cereus — Bacillus cereus (+),
B. megaterium — Bacillus megaterium (+),
B. spizizenii — Bacillus spizizenii (–),
B. subtilis — Bacillus subtilis (+),
C. albicans — Candida albicans (f ),
C. auris — Candida auris (f ),
C. freundii — Citrobacter freundii (–),
C. jejuni — Campylobacter jejuni (–),
C. neoformans — Cryptococcus neoformans (f ),
C. parapsilosis — Candida parapsilosis (f ),
C. tropicalis — Candida tropicalis (f ),
E. coli — Escherichia coli (–),
E. faecalis — Enterococcus faecalis (+),
E. floccosum — Epidermophyton floccosum (f ),
F. oxysporum — Fusarium oxysporum (f ),
G. candidum — Geotrichum candidum (f ),
K. aerogenes — Klebsiella aerogenes (–),
K. pneumonia — Klebsiella pneumonia (–),
L. amazonensis — Leishmania amazonensis,
L. fermentum — Lactobacillus fermentum (+),
L. monocytogenes — Listeria monocytogenes (+),
M. canis — Microsporum canis (f ),
M. gypseum — Microsporum gypseum (f ),
M. luteus — Micrococcus luteus (+),
MRSA — methicillin-resistant Staphylococcus aureus,
N. gonorrhoeae — Neisseria gonorrhoeae (–),
P. aeruginosa — Pseudomonas aeruginosa (–),
P. desmolyticum — Pseudomonas desmolyticum,
P. mirabilis — Proteus mirabilis (–),
P. vulgaris — Proteus vulgaris (–),
R. botaticola — Rhizoctonia botaticola (f ),
R. mucilaginosa — Rhodotorula mucilaginosa (f ),
S. aureus — Staphylococcus aureus (+),
S. brasiliensis — Sporothrix brasiliensis (f ),
S. cerevisiae — Saccharomyces cerevisiae (f ),
S. enterica — Salmonella enterica (–),
S. epidermidis — Staphylococcus epidermidis (+),
S. marcescens — Serratia marcescens (–),
S. mutans — Streptococcus mutans (+),
S. pyogenes — Streptococcus pyogenes (+),
S. typhi — Salmonella typhi (–),
S. typhimurium — Salmonella typhimurium (–),
T. mentagrophytes — Тrichophyton mentagrophytes (f ),
T. rubrum — Trichophyton rubrum (f ),
V. cholerae — Vibro cholera (–),
X. campestris — Xanthomonas campestris (–)
References
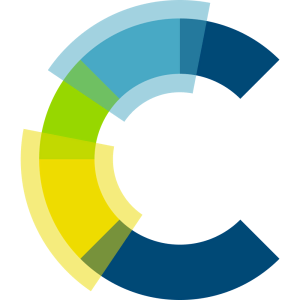
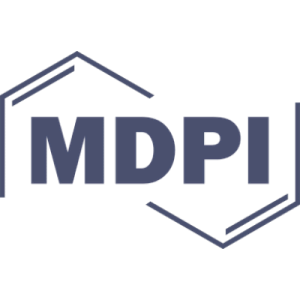
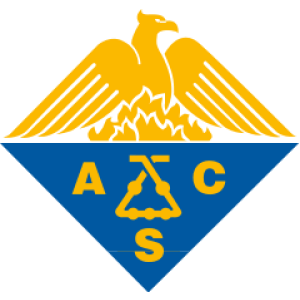
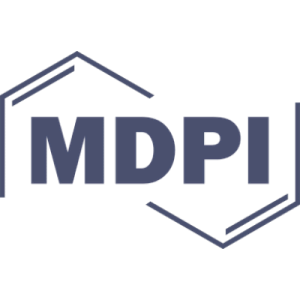
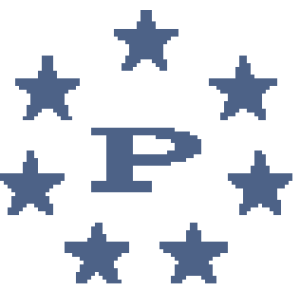
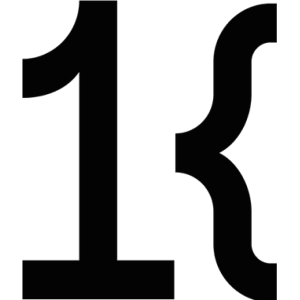
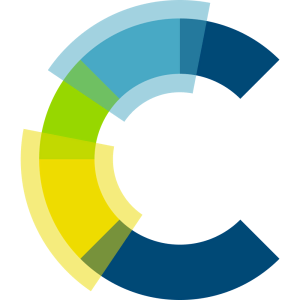
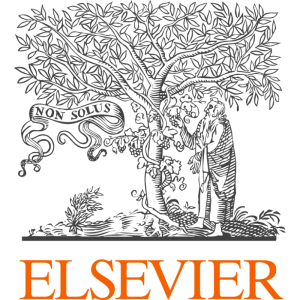
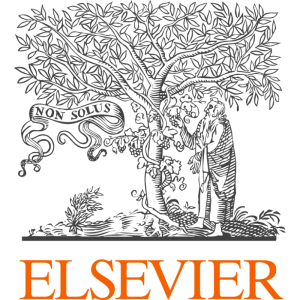
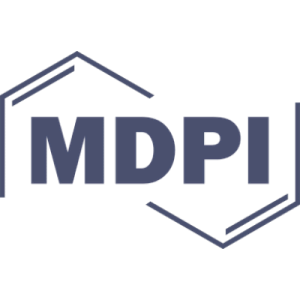
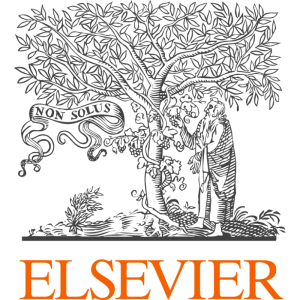
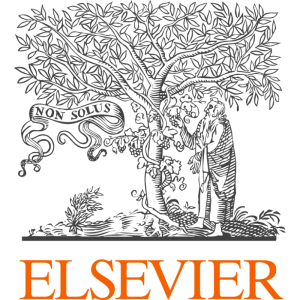
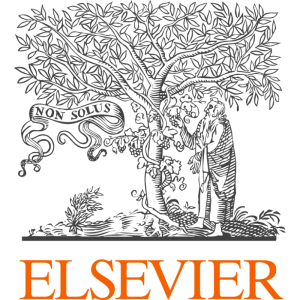
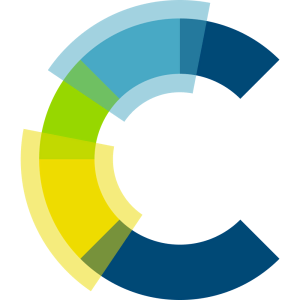
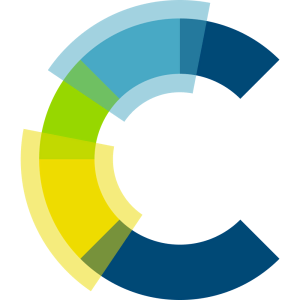
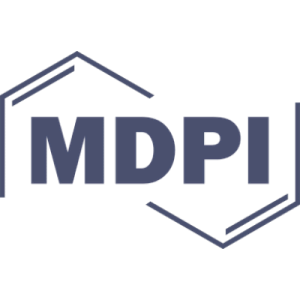
![Crystal Structure, Antitumour and Antibacterial Activity of Imidazo[1, 2‐α]pyridine Ligand Containing Palladium Complexes](/storage/images/resized/bRyGpdm98BkAUYiK1YFNpl5Z7hPu6Gd87gbIeuG3_small_thumb.webp)
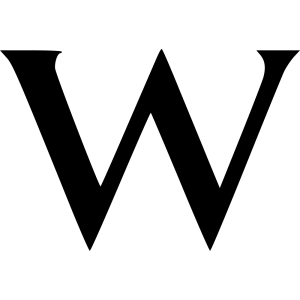
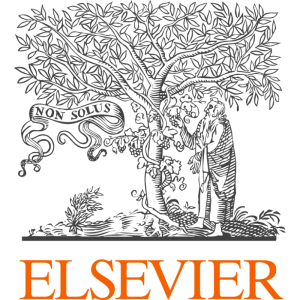
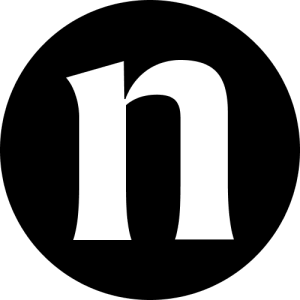
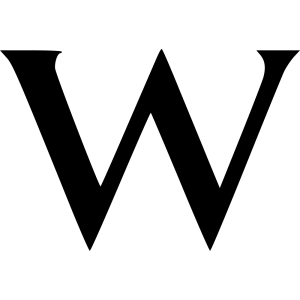
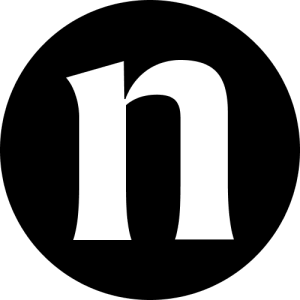
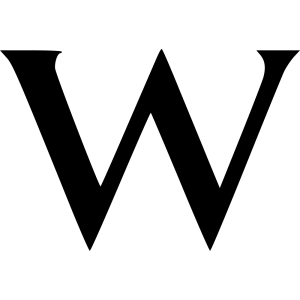
![Non-Linear Optical Property and Biological Assays of Therapeutic Potentials Under In Vitro Conditions of Pd(II), Ag(I) and Cu(II) Complexes of 5-Diethyl amino-2-({2-[(2-hydroxy-Benzylidene)-amino]-phenylimino}-methyl)-phenol](/storage/images/resized/MjH1ITP7lMYGxeqUZfkt2BnVLgjkk413jwBV97XX_small_thumb.webp)
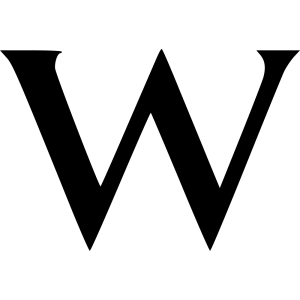
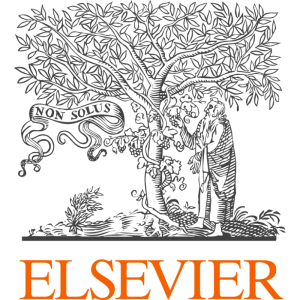
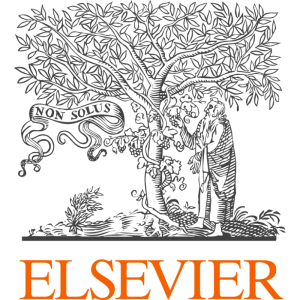
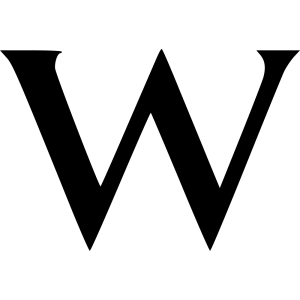
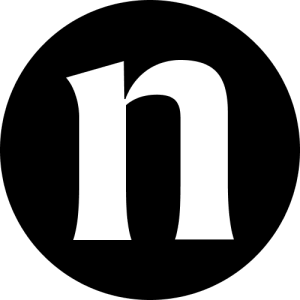
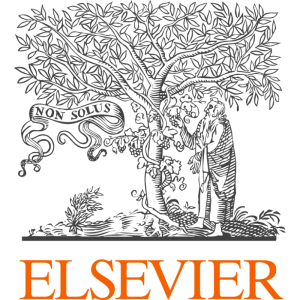
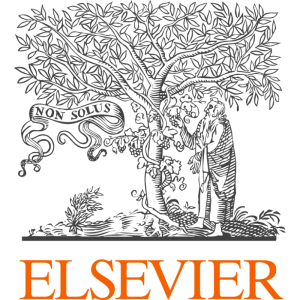
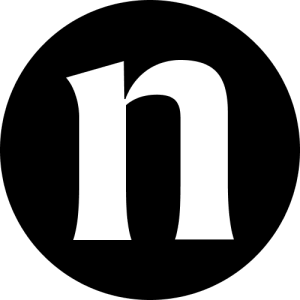
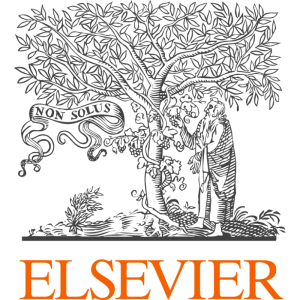
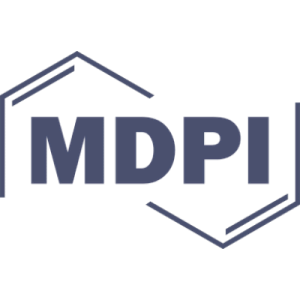
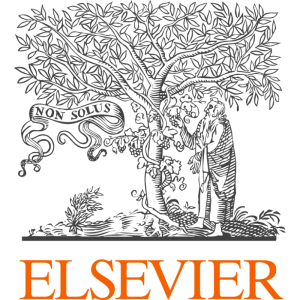
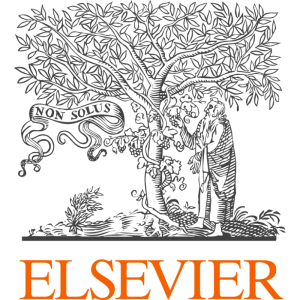
![Low-dimensional compounds containing bioactive ligands. Part XVII: Synthesis, structural, spectral and biological properties of hybrid organic-inorganic complexes based on [PdCl4]2− with derivatives of 8-hydroxyquinolinium](/storage/images/resized/GDnYOu1UpMMfMMRV6Aqle4H0YLLsraeD9IP9qScG_small_thumb.webp)
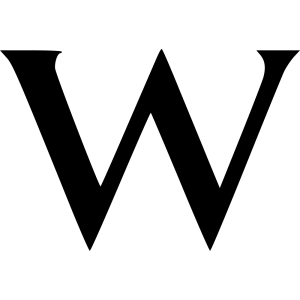
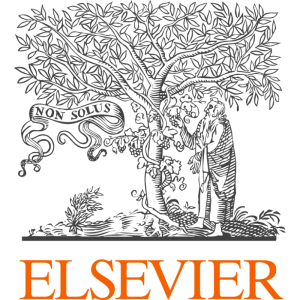
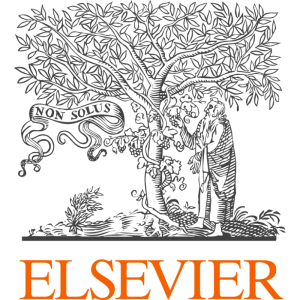
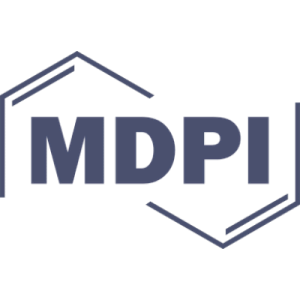
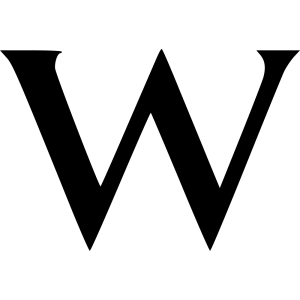
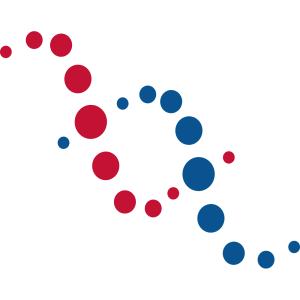
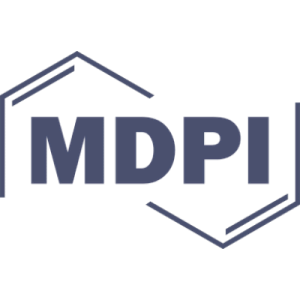
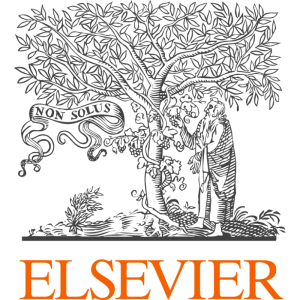
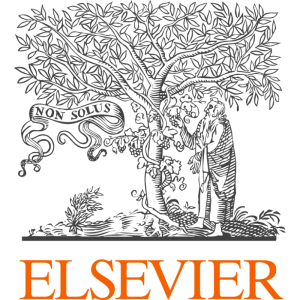
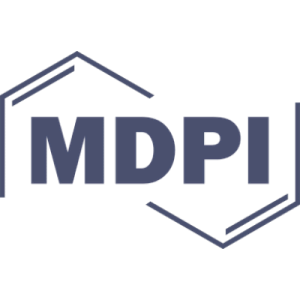
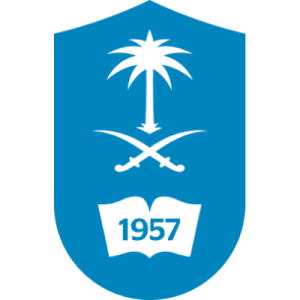
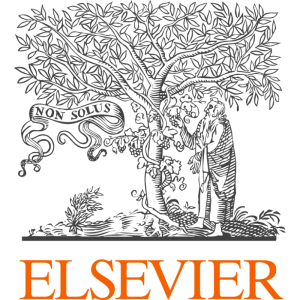
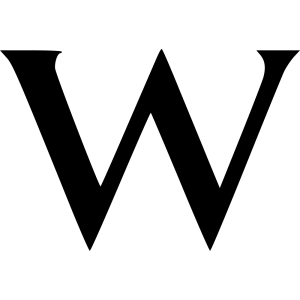
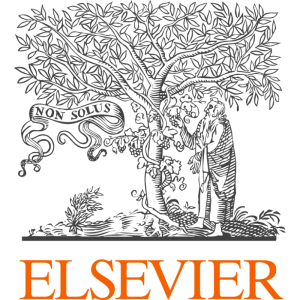
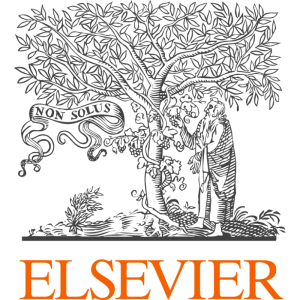
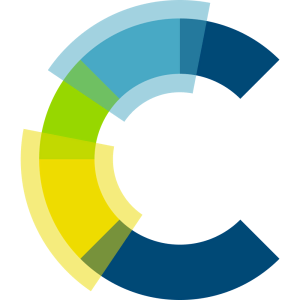
![Synthesis, spectral characterization, molecular modeling, antibacterial and antioxidant activities and stability study of binuclear Pd(II) and Ru(III) complexes with novel bis-[1-(2-[(2-hydroxynaphthalen-1-yl)methylidene]amino}ethyl)-1-ethyl-3-phenylthiourea] ligand: Application to detection of cholesterol](/storage/images/resized/GDnYOu1UpMMfMMRV6Aqle4H0YLLsraeD9IP9qScG_small_thumb.webp)
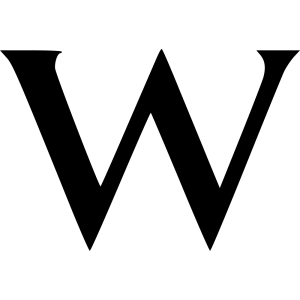
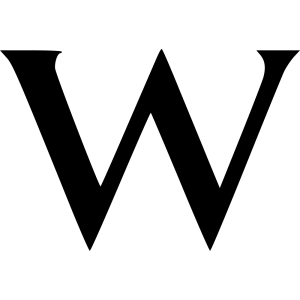
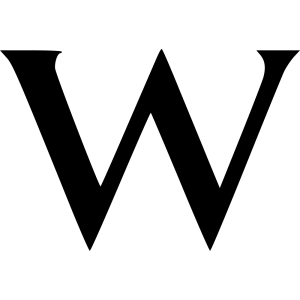
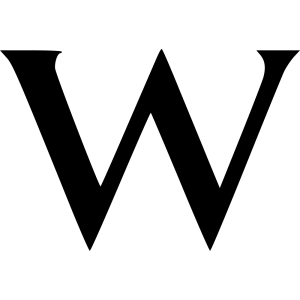
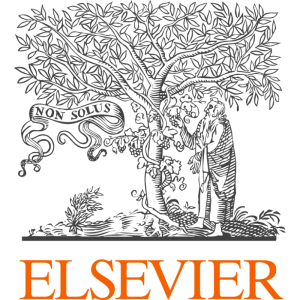
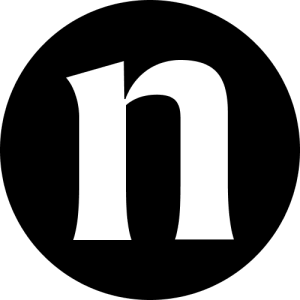
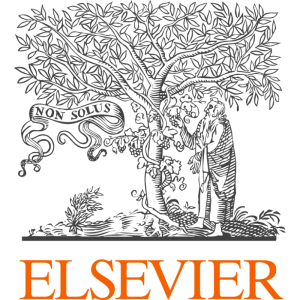
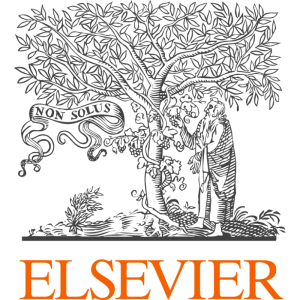
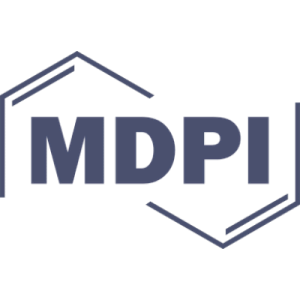
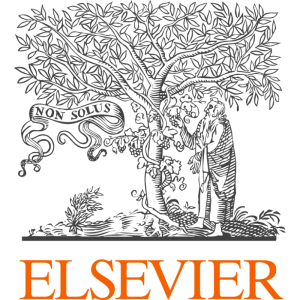
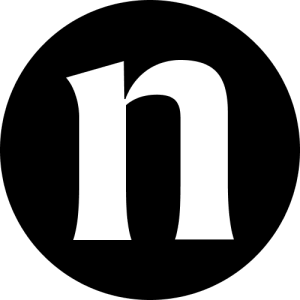
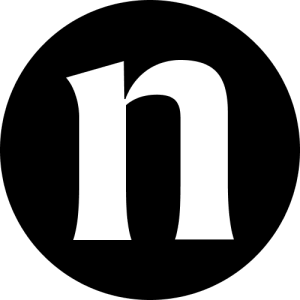
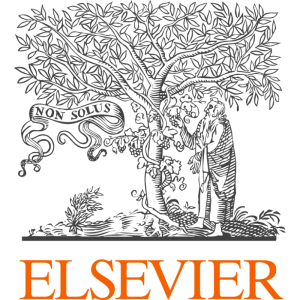
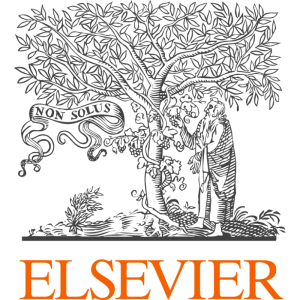
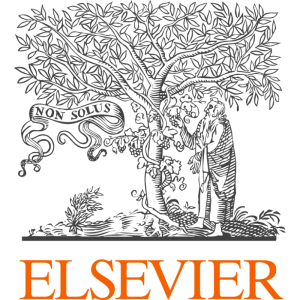
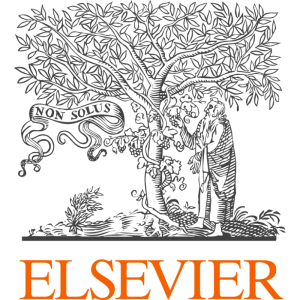
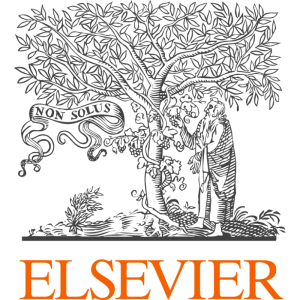
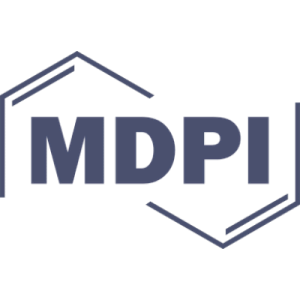
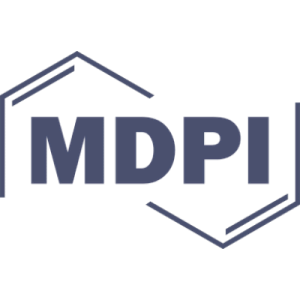
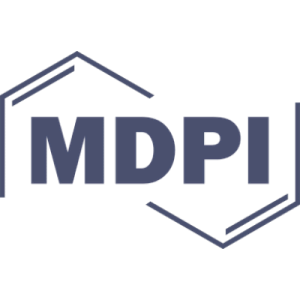
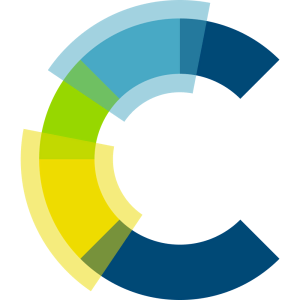
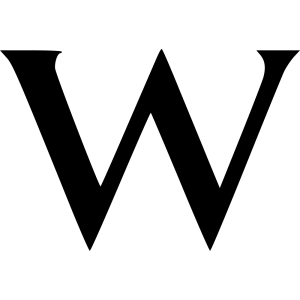
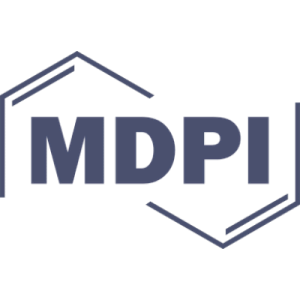
![Investigation of isomeric tetra-cationic porphyrin activity with peripheral [Pd(bpy)Cl]+ units by antimicrobial photodynamic therapy](/storage/images/resized/GDnYOu1UpMMfMMRV6Aqle4H0YLLsraeD9IP9qScG_small_thumb.webp)
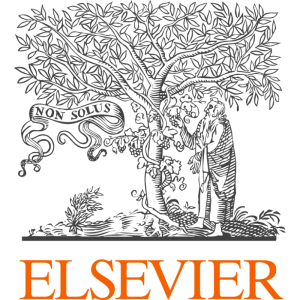
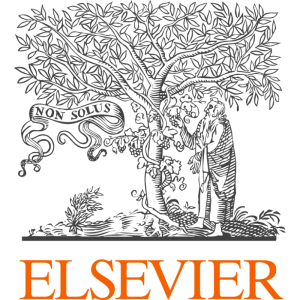
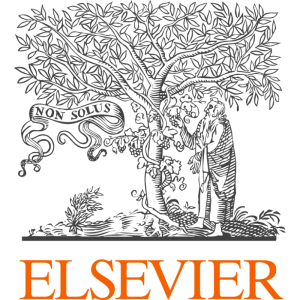
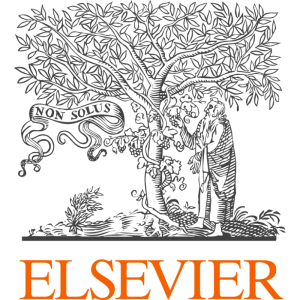
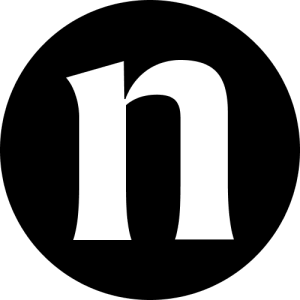
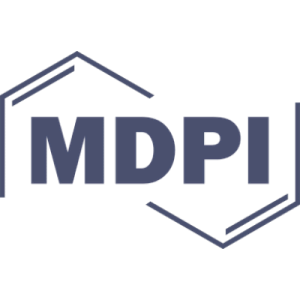
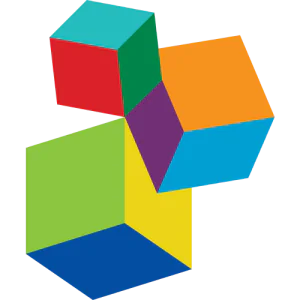
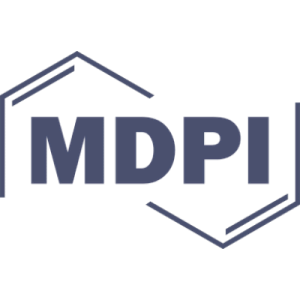
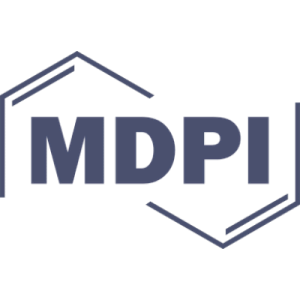
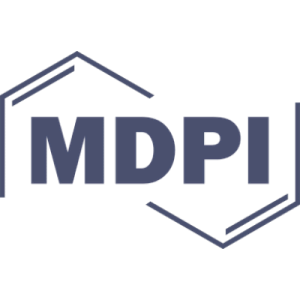
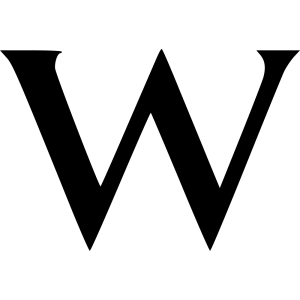
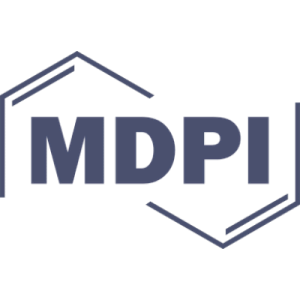